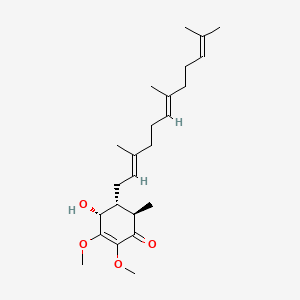
Antroquinonol
- Click on QUICK INQUIRY to receive a quote from our team of experts.
- With the quality product at a COMPETITIVE price, you can focus more on your research.
Overview
Description
Antroquinonol is a natural compound derived from the mycelium of the medicinal mushroom Antrodia camphorata, which is native to Taiwan. This compound has garnered significant attention due to its remarkable bioactivities, including anticancer, anti-inflammatory, and antioxidant properties . It is a member of the ubiquinone family and has shown potential in treating various diseases, particularly cancer .
Preparation Methods
Synthetic Routes and Reaction Conditions: Antroquinonol can be synthesized through a series of chemical reactions involving the precursor compounds. The synthesis typically involves the use of coenzyme Q0-induced fermentation, where the upregulated genes related to acetyl-CoA synthesis play a crucial role . The process includes the formation of the farnesyl tail precursor and the involvement of enzymes such as p-hydroxybenzoate polyprenyl transferase .
Industrial Production Methods: Industrial production of this compound involves the use of advanced chromatographic techniques for purification. UltraPerformance Convergence Chromatography (UPC2) and Preparative Supercritical Fluid Chromatography (Prep SFC) are employed to achieve high purity levels of the compound . These methods offer improved resolution and productivity compared to traditional reversed-phase liquid chromatography (RPLC) .
Chemical Reactions Analysis
Types of Reactions: Antroquinonol undergoes various chemical reactions, including oxidation, reduction, and substitution. These reactions are essential for its bioactivity and therapeutic potential.
Common Reagents and Conditions:
Oxidation: this compound can be oxidized using reagents such as hydrogen peroxide or potassium permanganate under controlled conditions.
Reduction: Reduction reactions can be carried out using reducing agents like sodium borohydride or lithium aluminum hydride.
Substitution: Substitution reactions often involve nucleophiles such as amines or thiols, which can replace specific functional groups in the compound.
Major Products Formed: The major products formed from these reactions include various derivatives of this compound, which may exhibit enhanced or modified bioactivities.
Scientific Research Applications
Mechanism of Action
Antroquinonol exerts its effects through multiple molecular targets and pathways:
AMP-activated protein kinase (AMPK) Activation: this compound activates AMPK, leading to the inhibition of the mammalian target of rapamycin (mTOR) pathway. This results in the suppression of cancer cell growth and proliferation.
Ras Pathway Inhibition: The compound inhibits the activity of protein farnesyltransferase, which is crucial for the post-translational modification of the Ras protein.
Nuclear Factor-kappa B (NF-κB) Inhibition: this compound suppresses the activation of NF-κB, reducing the production of pro-inflammatory cytokines and oxidative stress.
Comparison with Similar Compounds
Antroquinonol is unique due to its specific molecular structure and bioactivities. it shares similarities with other ubiquinone derivatives and terpenoids:
Similar Compounds:
Uniqueness: this compound’s ability to simultaneously induce apoptosis and autophagy in cancer cells, along with its multi-targeted mechanism of action, sets it apart from other similar compounds .
Properties
CAS No. |
1010081-09-0 |
---|---|
Molecular Formula |
C24H38O4 |
Molecular Weight |
390.6 g/mol |
IUPAC Name |
(4R,5R,6R)-4-hydroxy-2,3-dimethoxy-6-methyl-5-[(2E,6E)-3,7,11-trimethyldodeca-2,6,10-trienyl]cyclohex-2-en-1-one |
InChI |
InChI=1S/C24H38O4/c1-16(2)10-8-11-17(3)12-9-13-18(4)14-15-20-19(5)21(25)23(27-6)24(28-7)22(20)26/h10,12,14,19-20,22,26H,8-9,11,13,15H2,1-7H3/b17-12+,18-14+/t19-,20-,22-/m1/s1 |
InChI Key |
LJTSIMVOOOLKOL-FNRDIUJOSA-N |
SMILES |
CC1C(C(C(=C(C1=O)OC)OC)O)CC=C(C)CCC=C(C)CCC=C(C)C |
Isomeric SMILES |
C[C@@H]1[C@H]([C@H](C(=C(C1=O)OC)OC)O)C/C=C(\C)/CC/C=C(\C)/CCC=C(C)C |
Canonical SMILES |
CC1C(C(C(=C(C1=O)OC)OC)O)CC=C(C)CCC=C(C)CCC=C(C)C |
Appearance |
Solid powder |
Key on ui other cas no. |
1010081-09-0 |
Purity |
>98% (or refer to the Certificate of Analysis) |
shelf_life |
>2 years if stored properly |
solubility |
Soluble in DMSO |
storage |
Dry, dark and at 0 - 4 C for short term (days to weeks) or -20 C for long term (months to years). |
Synonyms |
(+)-antroquinonol A antroquinonol antroquinonol A antroquinonol D |
Origin of Product |
United States |
Retrosynthesis Analysis
AI-Powered Synthesis Planning: Our tool employs the Template_relevance Pistachio, Template_relevance Bkms_metabolic, Template_relevance Pistachio_ringbreaker, Template_relevance Reaxys, Template_relevance Reaxys_biocatalysis model, leveraging a vast database of chemical reactions to predict feasible synthetic routes.
One-Step Synthesis Focus: Specifically designed for one-step synthesis, it provides concise and direct routes for your target compounds, streamlining the synthesis process.
Accurate Predictions: Utilizing the extensive PISTACHIO, BKMS_METABOLIC, PISTACHIO_RINGBREAKER, REAXYS, REAXYS_BIOCATALYSIS database, our tool offers high-accuracy predictions, reflecting the latest in chemical research and data.
Strategy Settings
Precursor scoring | Relevance Heuristic |
---|---|
Min. plausibility | 0.01 |
Model | Template_relevance |
Template Set | Pistachio/Bkms_metabolic/Pistachio_ringbreaker/Reaxys/Reaxys_biocatalysis |
Top-N result to add to graph | 6 |
Feasible Synthetic Routes
Disclaimer and Information on In-Vitro Research Products
Please be aware that all articles and product information presented on BenchChem are intended solely for informational purposes. The products available for purchase on BenchChem are specifically designed for in-vitro studies, which are conducted outside of living organisms. In-vitro studies, derived from the Latin term "in glass," involve experiments performed in controlled laboratory settings using cells or tissues. It is important to note that these products are not categorized as medicines or drugs, and they have not received approval from the FDA for the prevention, treatment, or cure of any medical condition, ailment, or disease. We must emphasize that any form of bodily introduction of these products into humans or animals is strictly prohibited by law. It is essential to adhere to these guidelines to ensure compliance with legal and ethical standards in research and experimentation.