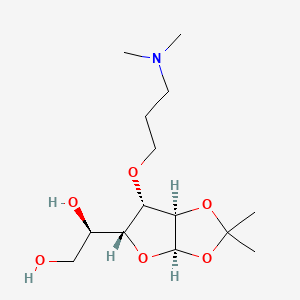
Therafectin
Overview
Description
Therafectin, also known as 1,2-O-isopropylidene-3-O-3′-(N’, N’-dimethylamino n-propyl)-D-gluco-furanose, hydrochloride, is a carbohydrate-based therapeutic compound. It has been studied for its anti-inflammatory, anti-proliferative, and immunomodulatory properties. This compound is particularly noted for its potential use in treating rheumatoid arthritis and other inflammatory conditions .
Mechanism of Action
Target of Action
Amiprilose primarily targets cyclooxygenase , an enzyme responsible for the synthesis of prostaglandins . Prostaglandins play a crucial role in inflammation, pain, and fever, making them a key target for anti-inflammatory drugs .
Mode of Action
Amiprilose acts by blocking the synthesis of prostaglandins . It achieves this by inhibiting cyclooxygenase, which converts arachidonic acid to cyclic endoperoxides, the precursors of prostaglandins . This inhibition disrupts the production of prostaglandins, thereby reducing inflammation, pain, and fever .
Biochemical Pathways
The primary biochemical pathway affected by Amiprilose is the prostaglandin synthesis pathway . By inhibiting cyclooxygenase, Amiprilose prevents the conversion of arachidonic acid to cyclic endoperoxides, effectively halting the production of prostaglandins . This has downstream effects on inflammation, pain, and fever, which are all mediated by prostaglandins .
Result of Action
Amiprilose has been shown to inhibit the proliferation of a number of hyperproliferative cell types, including psoriatic skin cells . In addition to its anti-inflammatory actions, Amiprilose also has analgesic, antipyretic, and platelet-inhibitory actions . These effects are likely due to its inhibition of prostaglandin synthesis .
Action Environment
The action of Amiprilose can be influenced by various environmental factors. For instance, the concentration of the drug can affect its efficacy and toxicity . In studies, concentrations of Amiprilose hydrochloride between 0.1 and 0.5% resulted in changes in fibroblast morphology, while concentrations greater than 5% were toxic . Thus, the dosage and administration of Amiprilose must be carefully controlled to ensure its safety and effectiveness.
Biochemical Analysis
Biochemical Properties
Amiprilose plays a significant role in biochemical reactions by interacting with various enzymes, proteins, and other biomolecules. It has been shown to inhibit the synthesis of prostaglandins by blocking the activity of cyclooxygenase enzymes, which are crucial in the inflammatory response . Additionally, Amiprilose interacts with cytokines, modulating their regulatory effects in vitro . These interactions highlight the compound’s potential as an anti-inflammatory and immunomodulatory agent.
Cellular Effects
Amiprilose has been observed to affect various cell types and cellular processes. In human skin equivalents, Amiprilose inhibits the proliferation of hyperproliferative cell types, such as psoriatic skin cells . It also influences cell signaling pathways, gene expression, and cellular metabolism by modulating the activity of enzymes like phospholipase A2, which plays a role in eicosanoid synthesis . These effects underscore the compound’s potential in regulating cellular functions and treating hyperproliferative disorders.
Molecular Mechanism
The molecular mechanism of Amiprilose involves its binding interactions with biomolecules and enzyme inhibition. Amiprilose exerts its effects by inhibiting cyclooxygenase enzymes, thereby reducing the synthesis of prostaglandins . This inhibition leads to decreased inflammation and pain. Additionally, Amiprilose modulates gene expression by affecting the activity of transcription factors involved in the inflammatory response . These molecular interactions contribute to the compound’s therapeutic potential.
Temporal Effects in Laboratory Settings
In laboratory settings, the effects of Amiprilose change over time. Studies have shown that Amiprilose remains stable at concentrations of 0.1% (wt/vol) and lower, with no toxic effects on fibroblasts and keratinocytes . Higher concentrations can lead to changes in cell morphology and toxicity. Long-term effects of Amiprilose include modulation of cytokine production and eicosanoid synthesis, which can influence cellular functions over extended periods .
Dosage Effects in Animal Models
The effects of Amiprilose vary with different dosages in animal models. At lower dosages, Amiprilose has been shown to be non-toxic and effective in modulating immune responses . Higher dosages can lead to adverse effects, such as changes in cell morphology and toxicity. These findings highlight the importance of determining optimal dosages for therapeutic applications to minimize potential side effects.
Metabolic Pathways
Amiprilose is involved in several metabolic pathways, including the regulation of eicosanoid synthesis. It interacts with enzymes such as phospholipase A2, which plays a role in the production of eicosanoids . These interactions can affect metabolic flux and metabolite levels, influencing the overall metabolic state of cells. Understanding these pathways is crucial for elucidating the compound’s therapeutic mechanisms.
Transport and Distribution
Amiprilose is transported and distributed within cells and tissues through interactions with transporters and binding proteins. It is known to interact with solute carrier (SLC) transporters, which facilitate its movement across cell membranes . The distribution of Amiprilose is influenced by factors such as blood flow, tissue binding, and regional pH, affecting its localization and accumulation within tissues .
Subcellular Localization
The subcellular localization of Amiprilose is determined by targeting signals and post-translational modifications. Amiprilose has been observed to localize in specific cellular compartments, such as the cytoplasm and nucleus . These localizations can influence its activity and function, as the compound interacts with various biomolecules within these compartments. Understanding the subcellular distribution of Amiprilose is essential for elucidating its therapeutic mechanisms.
Preparation Methods
Synthetic Routes and Reaction Conditions
Therafectin is synthesized through a multi-step process starting from D-glucose. The key steps involve the protection of hydroxyl groups, introduction of the dimethylamino propyl group, and formation of the isopropylidene acetal. The reaction conditions typically involve the use of acid catalysts and protective groups to ensure selective reactions at specific hydroxyl sites .
Industrial Production Methods
Industrial production of this compound involves large-scale synthesis using bioreactors and immobilized enzymes. This method ensures high yield and purity of the final product. The process is optimized to minimize the use of hazardous reagents and reduce environmental impact .
Chemical Reactions Analysis
Types of Reactions
Therafectin undergoes various chemical reactions, including:
Oxidation: In the presence of oxidizing agents, this compound can form corresponding ketones or aldehydes.
Reduction: Reduction reactions can convert the compound into its alcohol derivatives.
Substitution: Nucleophilic substitution reactions can introduce different functional groups into the molecule.
Common Reagents and Conditions
Oxidation: Common oxidizing agents include potassium permanganate and chromium trioxide.
Reduction: Sodium borohydride and lithium aluminum hydride are frequently used reducing agents.
Substitution: Reagents such as alkyl halides and acyl chlorides are used under basic or acidic conditions.
Major Products
The major products formed from these reactions include various derivatives of this compound with modified functional groups, which can be further utilized in different applications .
Scientific Research Applications
Therafectin has a wide range of scientific research applications:
Chemistry: Used as a model compound for studying carbohydrate-based reactions and mechanisms.
Biology: Investigated for its role in modulating immune responses and cell signaling pathways.
Medicine: Explored as a potential therapeutic agent for treating inflammatory diseases, including rheumatoid arthritis.
Industry: Utilized in the development of new pharmaceuticals and as a reference compound in quality control processes
Comparison with Similar Compounds
Similar Compounds
Isoprinosine: Another immunomodulatory agent with antiviral properties.
Levamisole: Used as an immunomodulator and antihelminthic agent.
Muramyl Dipeptide: Known for its immune-stimulating properties.
Uniqueness of Therafectin
This compound stands out due to its carbohydrate-based structure, which provides unique flexibility and specificity in modulating immune responses. Unlike other similar compounds, this compound is orally available and has shown promising results in clinical trials for treating chronic inflammatory conditions .
Properties
CAS No. |
56824-20-5 |
---|---|
Molecular Formula |
C14H27NO6 |
Molecular Weight |
305.37 g/mol |
IUPAC Name |
(1R)-1-[(3aR,5R,6S,6aR)-6-[3-(dimethylamino)propoxy]-2,2-dimethyl-3a,5,6,6a-tetrahydrofuro[2,3-d][1,3]dioxol-5-yl]ethane-1,2-diol |
InChI |
InChI=1S/C14H27NO6/c1-14(2)20-12-11(18-7-5-6-15(3)4)10(9(17)8-16)19-13(12)21-14/h9-13,16-17H,5-8H2,1-4H3/t9-,10-,11+,12-,13-/m1/s1 |
InChI Key |
YXBQLONCIPUQKO-UJPOAAIJSA-N |
SMILES |
CC1(OC2C(C(OC2O1)C(CO)O)OCCCN(C)C)C.Cl |
Isomeric SMILES |
CC1(O[C@@H]2[C@H]([C@H](O[C@@H]2O1)[C@@H](CO)O)OCCCN(C)C)C |
Canonical SMILES |
CC1(OC2C(C(OC2O1)C(CO)O)OCCCN(C)C)C |
Appearance |
Solid powder |
Purity |
>98% (or refer to the Certificate of Analysis) |
Related CAS |
60414-06-4 (hydrochloride) |
shelf_life |
>3 years if stored properly |
solubility |
Soluble in DMSO |
storage |
Dry, dark and at 0 - 4 C for short term (days to weeks) or -20 C for long term (months to years). |
Synonyms |
1,2-O-isopropylidene-3-O-3'-(N',N'-dimethylamino-N-propyl)-D-glucofuranose amiprilose amiprilose hydrochloride amprilose SM 1213 SM-1213 Therafectin |
Origin of Product |
United States |
Retrosynthesis Analysis
AI-Powered Synthesis Planning: Our tool employs the Template_relevance Pistachio, Template_relevance Bkms_metabolic, Template_relevance Pistachio_ringbreaker, Template_relevance Reaxys, Template_relevance Reaxys_biocatalysis model, leveraging a vast database of chemical reactions to predict feasible synthetic routes.
One-Step Synthesis Focus: Specifically designed for one-step synthesis, it provides concise and direct routes for your target compounds, streamlining the synthesis process.
Accurate Predictions: Utilizing the extensive PISTACHIO, BKMS_METABOLIC, PISTACHIO_RINGBREAKER, REAXYS, REAXYS_BIOCATALYSIS database, our tool offers high-accuracy predictions, reflecting the latest in chemical research and data.
Strategy Settings
Precursor scoring | Relevance Heuristic |
---|---|
Min. plausibility | 0.01 |
Model | Template_relevance |
Template Set | Pistachio/Bkms_metabolic/Pistachio_ringbreaker/Reaxys/Reaxys_biocatalysis |
Top-N result to add to graph | 6 |
Feasible Synthetic Routes
Disclaimer and Information on In-Vitro Research Products
Please be aware that all articles and product information presented on BenchChem are intended solely for informational purposes. The products available for purchase on BenchChem are specifically designed for in-vitro studies, which are conducted outside of living organisms. In-vitro studies, derived from the Latin term "in glass," involve experiments performed in controlled laboratory settings using cells or tissues. It is important to note that these products are not categorized as medicines or drugs, and they have not received approval from the FDA for the prevention, treatment, or cure of any medical condition, ailment, or disease. We must emphasize that any form of bodily introduction of these products into humans or animals is strictly prohibited by law. It is essential to adhere to these guidelines to ensure compliance with legal and ethical standards in research and experimentation.