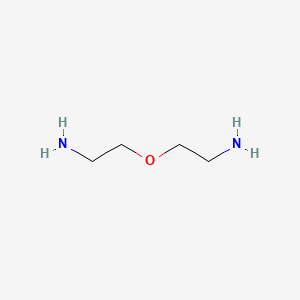
Ethanamine, 2,2'-oxybis-
Overview
Description
Amino-PEG1-amine is a PEG derivative containing two amino groups. The amino groups are reactive with carboxylic acids, activated NHS esters, carbonyls (ketone, aldehyde) etc. PEG Linkers may be useful in the development of antibody drug conjugates and drug delivery methods.
Mechanism of Action
Mode of Action
The mode of action of 2,2’-Oxydiethanamine is not well-understood due to the lack of specific studies on this compound. Given its structure, it may interact with its targets through hydrogen bonding or electrostatic interactions. The presence of two amine groups could allow it to act as a linker molecule in certain biochemical reactions .
Pharmacokinetics
The pharmacokinetics of 2,2’-Oxydiethanamine, including its absorption, distribution, metabolism, and excretion (ADME) properties, are not well-studied. Its water solubility suggests it could be readily absorbed and distributed in the body. The compound’s metabolism and excretion would likely involve enzymatic processes, but specific details are currently unknown .
Result of Action
The specific molecular and cellular effects of 2,2’-Oxydiethanamine’s action are not well-documented. Given its chemical structure, it could potentially influence a variety of cellular processes. More research is needed to understand the specific effects of this compound .
Action Environment
Environmental factors such as pH, temperature, and the presence of other molecules could influence the action, efficacy, and stability of 2,2’-Oxydiethanamine. For example, its reactivity could be affected by pH due to the presence of amine groups. Similarly, temperature could influence the rate of any reactions it participates in .
Biochemical Analysis
Biochemical Properties
2,2’-Oxydiethanamine plays a crucial role in biochemical reactions, particularly in the synthesis of macrocyclic bisimines . It interacts with various enzymes and proteins, facilitating the formation of complex molecular structures. The compound’s ability to form stable complexes with metal ions makes it valuable in coordination chemistry and catalysis. Additionally, 2,2’-Oxydiethanamine can act as a chelating agent, binding to metal ions and enhancing their solubility and reactivity in biochemical processes .
Cellular Effects
2,2’-Oxydiethanamine influences various cellular processes, including cell signaling pathways, gene expression, and cellular metabolism. It has been observed to modulate the activity of specific enzymes involved in metabolic pathways, thereby affecting the overall metabolic flux within cells . The compound’s interaction with cellular membranes and transport proteins also plays a role in regulating intracellular concentrations of essential ions and molecules .
Molecular Mechanism
At the molecular level, 2,2’-Oxydiethanamine exerts its effects through binding interactions with biomolecules, enzyme inhibition or activation, and changes in gene expression. The compound’s ability to form hydrogen bonds and coordinate with metal ions allows it to interact with various enzymes and proteins, altering their activity and stability . These interactions can lead to the activation or inhibition of specific biochemical pathways, ultimately influencing cellular function and metabolism .
Temporal Effects in Laboratory Settings
In laboratory settings, the effects of 2,2’-Oxydiethanamine can change over time due to its stability and degradation properties. The compound is relatively stable under standard laboratory conditions, but prolonged exposure to light and air can lead to its gradual degradation . Long-term studies have shown that 2,2’-Oxydiethanamine can have sustained effects on cellular function, particularly in in vitro and in vivo models .
Dosage Effects in Animal Models
The effects of 2,2’-Oxydiethanamine vary with different dosages in animal models. At low doses, the compound has been shown to enhance metabolic activity and improve cellular function . At high doses, it can exhibit toxic effects, including enzyme inhibition and disruption of cellular homeostasis . Threshold effects have been observed, indicating that the compound’s impact on cellular processes is dose-dependent .
Metabolic Pathways
2,2’-Oxydiethanamine is involved in several metabolic pathways, interacting with enzymes and cofactors that regulate metabolic flux and metabolite levels . The compound’s role in glycolysis and the tricarboxylic acid cycle has been well-documented, highlighting its importance in energy production and cellular metabolism . Additionally, 2,2’-Oxydiethanamine can influence the synthesis and degradation of various biomolecules, further impacting metabolic pathways .
Transport and Distribution
Within cells and tissues, 2,2’-Oxydiethanamine is transported and distributed through interactions with specific transporters and binding proteins . These interactions facilitate the compound’s localization and accumulation in particular cellular compartments, influencing its overall activity and function . The compound’s ability to cross cellular membranes and interact with intracellular targets is crucial for its biochemical effects .
Subcellular Localization
The subcellular localization of 2,2’-Oxydiethanamine is determined by targeting signals and post-translational modifications that direct it to specific compartments or organelles . The compound’s presence in the cytoplasm, mitochondria, and other organelles has been observed, indicating its involvement in various cellular processes . These localization patterns are essential for understanding the compound’s role in cellular function and metabolism .
Properties
IUPAC Name |
2-(2-aminoethoxy)ethanamine | |
---|---|---|
Source | PubChem | |
URL | https://pubchem.ncbi.nlm.nih.gov | |
Description | Data deposited in or computed by PubChem | |
InChI |
InChI=1S/C4H12N2O/c5-1-3-7-4-2-6/h1-6H2 | |
Source | PubChem | |
URL | https://pubchem.ncbi.nlm.nih.gov | |
Description | Data deposited in or computed by PubChem | |
InChI Key |
GXVUZYLYWKWJIM-UHFFFAOYSA-N | |
Source | PubChem | |
URL | https://pubchem.ncbi.nlm.nih.gov | |
Description | Data deposited in or computed by PubChem | |
Canonical SMILES |
C(COCCN)N | |
Source | PubChem | |
URL | https://pubchem.ncbi.nlm.nih.gov | |
Description | Data deposited in or computed by PubChem | |
Molecular Formula |
C4H12N2O | |
Source | PubChem | |
URL | https://pubchem.ncbi.nlm.nih.gov | |
Description | Data deposited in or computed by PubChem | |
DSSTOX Substance ID |
DTXSID7041914 | |
Record name | 2,2'-Oxybis(ethylamine) | |
Source | EPA DSSTox | |
URL | https://comptox.epa.gov/dashboard/DTXSID7041914 | |
Description | DSSTox provides a high quality public chemistry resource for supporting improved predictive toxicology. | |
Molecular Weight |
104.15 g/mol | |
Source | PubChem | |
URL | https://pubchem.ncbi.nlm.nih.gov | |
Description | Data deposited in or computed by PubChem | |
Physical Description |
Liquid | |
Record name | Ethanamine, 2,2'-oxybis- | |
Source | EPA Chemicals under the TSCA | |
URL | https://www.epa.gov/chemicals-under-tsca | |
Description | EPA Chemicals under the Toxic Substances Control Act (TSCA) collection contains information on chemicals and their regulations under TSCA, including non-confidential content from the TSCA Chemical Substance Inventory and Chemical Data Reporting. | |
CAS No. |
2752-17-2 | |
Record name | 2-Aminoethyl ether | |
Source | CAS Common Chemistry | |
URL | https://commonchemistry.cas.org/detail?cas_rn=2752-17-2 | |
Description | CAS Common Chemistry is an open community resource for accessing chemical information. Nearly 500,000 chemical substances from CAS REGISTRY cover areas of community interest, including common and frequently regulated chemicals, and those relevant to high school and undergraduate chemistry classes. This chemical information, curated by our expert scientists, is provided in alignment with our mission as a division of the American Chemical Society. | |
Explanation | The data from CAS Common Chemistry is provided under a CC-BY-NC 4.0 license, unless otherwise stated. | |
Record name | Bis(2-aminoethyl) ether | |
Source | ChemIDplus | |
URL | https://pubchem.ncbi.nlm.nih.gov/substance/?source=chemidplus&sourceid=0002752172 | |
Description | ChemIDplus is a free, web search system that provides access to the structure and nomenclature authority files used for the identification of chemical substances cited in National Library of Medicine (NLM) databases, including the TOXNET system. | |
Record name | Ethanamine, 2,2'-oxybis- | |
Source | EPA Chemicals under the TSCA | |
URL | https://www.epa.gov/chemicals-under-tsca | |
Description | EPA Chemicals under the Toxic Substances Control Act (TSCA) collection contains information on chemicals and their regulations under TSCA, including non-confidential content from the TSCA Chemical Substance Inventory and Chemical Data Reporting. | |
Record name | 2,2'-Oxybis(ethylamine) | |
Source | EPA DSSTox | |
URL | https://comptox.epa.gov/dashboard/DTXSID7041914 | |
Description | DSSTox provides a high quality public chemistry resource for supporting improved predictive toxicology. | |
Record name | 2,2'-oxydi(ethylamine) | |
Source | European Chemicals Agency (ECHA) | |
URL | https://echa.europa.eu/substance-information/-/substanceinfo/100.018.541 | |
Description | The European Chemicals Agency (ECHA) is an agency of the European Union which is the driving force among regulatory authorities in implementing the EU's groundbreaking chemicals legislation for the benefit of human health and the environment as well as for innovation and competitiveness. | |
Explanation | Use of the information, documents and data from the ECHA website is subject to the terms and conditions of this Legal Notice, and subject to other binding limitations provided for under applicable law, the information, documents and data made available on the ECHA website may be reproduced, distributed and/or used, totally or in part, for non-commercial purposes provided that ECHA is acknowledged as the source: "Source: European Chemicals Agency, http://echa.europa.eu/". Such acknowledgement must be included in each copy of the material. ECHA permits and encourages organisations and individuals to create links to the ECHA website under the following cumulative conditions: Links can only be made to webpages that provide a link to the Legal Notice page. | |
Record name | BIS(2-AMINOETHYL) ETHER | |
Source | FDA Global Substance Registration System (GSRS) | |
URL | https://gsrs.ncats.nih.gov/ginas/app/beta/substances/FFW1773ZZ6 | |
Description | The FDA Global Substance Registration System (GSRS) enables the efficient and accurate exchange of information on what substances are in regulated products. Instead of relying on names, which vary across regulatory domains, countries, and regions, the GSRS knowledge base makes it possible for substances to be defined by standardized, scientific descriptions. | |
Explanation | Unless otherwise noted, the contents of the FDA website (www.fda.gov), both text and graphics, are not copyrighted. They are in the public domain and may be republished, reprinted and otherwise used freely by anyone without the need to obtain permission from FDA. Credit to the U.S. Food and Drug Administration as the source is appreciated but not required. | |
Retrosynthesis Analysis
AI-Powered Synthesis Planning: Our tool employs the Template_relevance Pistachio, Template_relevance Bkms_metabolic, Template_relevance Pistachio_ringbreaker, Template_relevance Reaxys, Template_relevance Reaxys_biocatalysis model, leveraging a vast database of chemical reactions to predict feasible synthetic routes.
One-Step Synthesis Focus: Specifically designed for one-step synthesis, it provides concise and direct routes for your target compounds, streamlining the synthesis process.
Accurate Predictions: Utilizing the extensive PISTACHIO, BKMS_METABOLIC, PISTACHIO_RINGBREAKER, REAXYS, REAXYS_BIOCATALYSIS database, our tool offers high-accuracy predictions, reflecting the latest in chemical research and data.
Strategy Settings
Precursor scoring | Relevance Heuristic |
---|---|
Min. plausibility | 0.01 |
Model | Template_relevance |
Template Set | Pistachio/Bkms_metabolic/Pistachio_ringbreaker/Reaxys/Reaxys_biocatalysis |
Top-N result to add to graph | 6 |
Feasible Synthetic Routes
Disclaimer and Information on In-Vitro Research Products
Please be aware that all articles and product information presented on BenchChem are intended solely for informational purposes. The products available for purchase on BenchChem are specifically designed for in-vitro studies, which are conducted outside of living organisms. In-vitro studies, derived from the Latin term "in glass," involve experiments performed in controlled laboratory settings using cells or tissues. It is important to note that these products are not categorized as medicines or drugs, and they have not received approval from the FDA for the prevention, treatment, or cure of any medical condition, ailment, or disease. We must emphasize that any form of bodily introduction of these products into humans or animals is strictly prohibited by law. It is essential to adhere to these guidelines to ensure compliance with legal and ethical standards in research and experimentation.