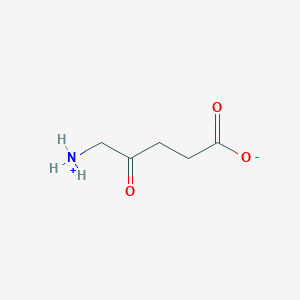
5-Aminolevulinic acid
Overview
Description
Aminolevulinic acid is a non-proteinogenic amino acid that plays a crucial role in the biosynthesis of tetrapyrrole compounds such as heme, chlorophyll, and vitamin B12 . It is an endogenous compound found in various organisms, including animals, plants, fungi, and bacteria . Aminolevulinic acid is widely used in medical, agricultural, and industrial applications due to its unique properties .
Mechanism of Action
Target of Action
5-Aminolevulinic acid (ALA) is a precursor of protoporphyrin IX (PpIX), which is involved in the synthesis of heme, a vital component of hemoglobin . The primary target of ALA is the mitochondrion , where it is metabolized to PpIX .
Mode of Action
ALA’s mode of action involves its metabolic conversion to PpIX, which accumulates in the skin where ALA has been applied . This conversion is part of the heme biosynthesis pathway, where ALA is synthesized from succinyl-CoA and glycine . PpIX is a photosensitizer, meaning it can absorb light and transfer that energy to other molecules . This property is utilized in photodynamic therapy for the treatment of various conditions .
Biochemical Pathways
ALA is involved in the biosynthesis of tetrapyrrole compounds, such as heme, porphyrin, and chlorophyll . It can be biosynthesized through the C4 pathway, which uses succinyl-CoA and glycine as precursors, or through the C5 pathway, which uses glutamate as a precursor . After ALA biosynthesis, two ALA molecules are coalesced to form a pyrrol ring, called porphobilinogen (PBG); this reaction is catalyzed by ALA dehydratase (ALAD) .
Pharmacokinetics
The pharmacokinetics of ALA involve its absorption, distribution, metabolism, and excretion (ADME). After oral or intravenous administration, ALA has a terminal half-life of approximately 45 minutes . The oral bioavailability of ALA is approximately 60% . After intravesical administration, urine production is largely responsible for decreases in ALA concentration in the bladder, with less than 1% being absorbed into the systemic circulation .
Result of Action
The result of ALA’s action is the accumulation of PpIX, which can be used in photodynamic therapy for the treatment of various conditions, including actinic keratosis and glioma . PpIX, as a photosensitizer, can absorb light and transfer that energy to other molecules, causing a reaction that can kill cells . This property is particularly useful in the treatment of cancer, where the goal is to selectively kill cancer cells .
Action Environment
The action of ALA can be influenced by various environmental factors. For example, in plants, ALA enhances the ability to cope with various stresses such as drought stress, salt stress, UV-B stress, and extreme temperature stress by regulating photosynthesis, osmoregulation, antioxidant capacity, and nitrogen assimilation . In the context of cancer treatment, the efficacy of ALA can be influenced by factors such as the presence of light (for photodynamic therapy) and the specific characteristics of the tumor cells .
Biochemical Analysis
Biochemical Properties
5-Aminolevulinic acid plays a significant role in biochemical reactions. It is the key precursor for the biosynthesis of tetrapyrrole compounds . As the precursor of heme, chlorophylls, and vitamin B12, this compound significantly affects cell growth and metabolic flux .
Cellular Effects
This compound has various effects on different types of cells and cellular processes. For instance, it has been reported that this compound can promote aerobic energy metabolism, especially cytochrome c oxidase activity . It also influences cell function by affecting ATP levels in cells .
Molecular Mechanism
At the molecular level, this compound exerts its effects through a series of transformations. In humans, this compound, after being biosynthesized, goes through a series of transformations in the cytosol and finally gets converted to Protoporphyrin IX inside the mitochondria . This protoporphyrin molecule chelates with iron in the presence of the enzyme ferrochelatase to produce Heme .
Temporal Effects in Laboratory Settings
In laboratory settings, the effects of this compound can change over time. For instance, it has been observed that this compound can promote the regulation of the whole process of photosynthesis . It also induces plant preventive and protective systems through the NO/H2O2 signaling network .
Dosage Effects in Animal Models
The effects of this compound can vary with different dosages in animal models. For instance, in rodent models, administration of a high dose of this compound (30 - 100 mg/kg) has been reported to induce heme oxygenase-1, which can prevent renal ischemia-reperfusion injury .
Metabolic Pathways
This compound is involved in several metabolic pathways. It is the first compound in the porphyrin synthesis pathway, which leads to heme in mammals, as well as chlorophyll in plants . It is also involved in the C4 and C5 pathways related to heme biosynthesis in microorganisms .
Transport and Distribution
This compound is transported and distributed within cells and tissues. For instance, it has been demonstrated that this compound uses the intestinal and renal apical peptide transporters for entering into epithelial cells .
Subcellular Localization
The subcellular localization of this compound and its effects on activity or function are significant. For instance, in Arabidopsis, it has been found that this compound is a precursor of all tetrapyrroles such as chlorophyll, heme, and siroheme, and is involved in the regulation of the whole process of photosynthesis . In another study, it was found that this compound-induced Protoporphyrin IX was primarily localized in the endoplasmic reticulum and mitochondria .
Preparation Methods
Synthetic Routes and Reaction Conditions: Aminolevulinic acid can be synthesized through chemical and biological methods. The chemical synthesis involves the condensation of succinyl-CoA and glycine, catalyzed by the enzyme aminolevulinic acid synthase . This reaction occurs in the mitochondria of non-photosynthetic eukaryotes .
Industrial Production Methods: Microbial production of aminolevulinic acid has gained attention due to its higher yields and eco-friendly nature compared to chemical synthesis . Photosynthetic bacteria and engineered microbial strains are commonly used for industrial production . The process involves optimizing key enzymes and metabolic pathways to enhance the yield of aminolevulinic acid .
Chemical Reactions Analysis
Types of Reactions: Aminolevulinic acid undergoes various chemical reactions, including oxidation, reduction, and substitution .
Common Reagents and Conditions:
Oxidation: Aminolevulinic acid can be oxidized to form porphobilinogen, a precursor in the heme biosynthesis pathway.
Reduction: It can be reduced to form different derivatives used in photodynamic therapy.
Substitution: Aminolevulinic acid can undergo substitution reactions to form various esters and amides.
Major Products: The major products formed from these reactions include porphobilinogen, protoporphyrin IX, and various esters used in medical applications .
Scientific Research Applications
Aminolevulinic acid has a wide range of applications in scientific research:
Comparison with Similar Compounds
Aminolevulinic acid is unique due to its role as a precursor in the biosynthesis of tetrapyrrole compounds . Similar compounds include:
Porphobilinogen: Another intermediate in the heme biosynthesis pathway.
Protoporphyrin IX: A direct product of aminolevulinic acid metabolism.
Methyl aminolevulinate: A derivative used in photodynamic therapy.
Aminolevulinic acid stands out due to its versatility and wide range of applications in various fields .
Properties
IUPAC Name |
5-amino-4-oxopentanoic acid | |
---|---|---|
Source | PubChem | |
URL | https://pubchem.ncbi.nlm.nih.gov | |
Description | Data deposited in or computed by PubChem | |
InChI |
InChI=1S/C5H9NO3/c6-3-4(7)1-2-5(8)9/h1-3,6H2,(H,8,9) | |
Source | PubChem | |
URL | https://pubchem.ncbi.nlm.nih.gov | |
Description | Data deposited in or computed by PubChem | |
InChI Key |
ZGXJTSGNIOSYLO-UHFFFAOYSA-N | |
Source | PubChem | |
URL | https://pubchem.ncbi.nlm.nih.gov | |
Description | Data deposited in or computed by PubChem | |
Canonical SMILES |
C(CC(=O)O)C(=O)CN | |
Source | PubChem | |
URL | https://pubchem.ncbi.nlm.nih.gov | |
Description | Data deposited in or computed by PubChem | |
Molecular Formula |
C5H9NO3 | |
Source | PubChem | |
URL | https://pubchem.ncbi.nlm.nih.gov | |
Description | Data deposited in or computed by PubChem | |
DSSTOX Substance ID |
DTXSID8048490 | |
Record name | Aminolevulinic acid | |
Source | EPA DSSTox | |
URL | https://comptox.epa.gov/dashboard/DTXSID8048490 | |
Description | DSSTox provides a high quality public chemistry resource for supporting improved predictive toxicology. | |
Molecular Weight |
131.13 g/mol | |
Source | PubChem | |
URL | https://pubchem.ncbi.nlm.nih.gov | |
Description | Data deposited in or computed by PubChem | |
Physical Description |
Solid | |
Record name | 5-Aminolevulinic acid | |
Source | Human Metabolome Database (HMDB) | |
URL | http://www.hmdb.ca/metabolites/HMDB0001149 | |
Description | The Human Metabolome Database (HMDB) is a freely available electronic database containing detailed information about small molecule metabolites found in the human body. | |
Explanation | HMDB is offered to the public as a freely available resource. Use and re-distribution of the data, in whole or in part, for commercial purposes requires explicit permission of the authors and explicit acknowledgment of the source material (HMDB) and the original publication (see the HMDB citing page). We ask that users who download significant portions of the database cite the HMDB paper in any resulting publications. | |
Solubility |
18.6 [ug/mL] (The mean of the results at pH 7.4), Very soluble, 1000 mg/mL at 25 °C | |
Record name | SID56322917 | |
Source | Burnham Center for Chemical Genomics | |
URL | https://pubchem.ncbi.nlm.nih.gov/bioassay/1996#section=Data-Table | |
Description | Aqueous solubility in buffer at pH 7.4 | |
Record name | Aminolevulinic acid | |
Source | DrugBank | |
URL | https://www.drugbank.ca/drugs/DB00855 | |
Description | The DrugBank database is a unique bioinformatics and cheminformatics resource that combines detailed drug (i.e. chemical, pharmacological and pharmaceutical) data with comprehensive drug target (i.e. sequence, structure, and pathway) information. | |
Explanation | Creative Common's Attribution-NonCommercial 4.0 International License (http://creativecommons.org/licenses/by-nc/4.0/legalcode) | |
Record name | 5-Aminolevulinic acid | |
Source | Human Metabolome Database (HMDB) | |
URL | http://www.hmdb.ca/metabolites/HMDB0001149 | |
Description | The Human Metabolome Database (HMDB) is a freely available electronic database containing detailed information about small molecule metabolites found in the human body. | |
Explanation | HMDB is offered to the public as a freely available resource. Use and re-distribution of the data, in whole or in part, for commercial purposes requires explicit permission of the authors and explicit acknowledgment of the source material (HMDB) and the original publication (see the HMDB citing page). We ask that users who download significant portions of the database cite the HMDB paper in any resulting publications. | |
Mechanism of Action |
According to the presumed mechanism of action, photosensitization following application of aminolevulinic acid (ALA) topical solution occurs through the metabolic conversion of ALA to protoporphyrin IX (PpIX), which accumulates in the skin to which aminolevulinic acid has been applied. When exposed to light of appropriate wavelength and energy, the accumulated PpIX produces a photodynamic reaction, a cytotoxic process dependent upon the simultaneous presence of light and oxygen. The absorption of light results in an excited state of the porphyrin molecule, and subsequent spin transfer from PpIX to molecular oxygen generates singlet oxygen, which can further react to form superoxide and hydroxyl radicals. Photosensitization of actinic (solar) keratosis lesions using aminolevulinic acid, plus illumination with the BLU-UTM Blue Light Photodynamic Therapy Illuminator (BLU-U), is the basis for aminolevulinic acid photodynamic therapy (PDT). | |
Record name | Aminolevulinic acid | |
Source | DrugBank | |
URL | https://www.drugbank.ca/drugs/DB00855 | |
Description | The DrugBank database is a unique bioinformatics and cheminformatics resource that combines detailed drug (i.e. chemical, pharmacological and pharmaceutical) data with comprehensive drug target (i.e. sequence, structure, and pathway) information. | |
Explanation | Creative Common's Attribution-NonCommercial 4.0 International License (http://creativecommons.org/licenses/by-nc/4.0/legalcode) | |
CAS No. |
106-60-5 | |
Record name | 5-Aminolevulinic acid | |
Source | CAS Common Chemistry | |
URL | https://commonchemistry.cas.org/detail?cas_rn=106-60-5 | |
Description | CAS Common Chemistry is an open community resource for accessing chemical information. Nearly 500,000 chemical substances from CAS REGISTRY cover areas of community interest, including common and frequently regulated chemicals, and those relevant to high school and undergraduate chemistry classes. This chemical information, curated by our expert scientists, is provided in alignment with our mission as a division of the American Chemical Society. | |
Explanation | The data from CAS Common Chemistry is provided under a CC-BY-NC 4.0 license, unless otherwise stated. | |
Record name | Aminolevulinic acid | |
Source | ChemIDplus | |
URL | https://pubchem.ncbi.nlm.nih.gov/substance/?source=chemidplus&sourceid=0000106605 | |
Description | ChemIDplus is a free, web search system that provides access to the structure and nomenclature authority files used for the identification of chemical substances cited in National Library of Medicine (NLM) databases, including the TOXNET system. | |
Record name | Aminolevulinic acid | |
Source | DrugBank | |
URL | https://www.drugbank.ca/drugs/DB00855 | |
Description | The DrugBank database is a unique bioinformatics and cheminformatics resource that combines detailed drug (i.e. chemical, pharmacological and pharmaceutical) data with comprehensive drug target (i.e. sequence, structure, and pathway) information. | |
Explanation | Creative Common's Attribution-NonCommercial 4.0 International License (http://creativecommons.org/licenses/by-nc/4.0/legalcode) | |
Record name | Aminolevulinic acid | |
Source | EPA DSSTox | |
URL | https://comptox.epa.gov/dashboard/DTXSID8048490 | |
Description | DSSTox provides a high quality public chemistry resource for supporting improved predictive toxicology. | |
Record name | AMINOLEVULINIC ACID | |
Source | FDA Global Substance Registration System (GSRS) | |
URL | https://gsrs.ncats.nih.gov/ginas/app/beta/substances/88755TAZ87 | |
Description | The FDA Global Substance Registration System (GSRS) enables the efficient and accurate exchange of information on what substances are in regulated products. Instead of relying on names, which vary across regulatory domains, countries, and regions, the GSRS knowledge base makes it possible for substances to be defined by standardized, scientific descriptions. | |
Explanation | Unless otherwise noted, the contents of the FDA website (www.fda.gov), both text and graphics, are not copyrighted. They are in the public domain and may be republished, reprinted and otherwise used freely by anyone without the need to obtain permission from FDA. Credit to the U.S. Food and Drug Administration as the source is appreciated but not required. | |
Record name | 5-Aminolevulinic acid | |
Source | Human Metabolome Database (HMDB) | |
URL | http://www.hmdb.ca/metabolites/HMDB0001149 | |
Description | The Human Metabolome Database (HMDB) is a freely available electronic database containing detailed information about small molecule metabolites found in the human body. | |
Explanation | HMDB is offered to the public as a freely available resource. Use and re-distribution of the data, in whole or in part, for commercial purposes requires explicit permission of the authors and explicit acknowledgment of the source material (HMDB) and the original publication (see the HMDB citing page). We ask that users who download significant portions of the database cite the HMDB paper in any resulting publications. | |
Melting Point |
156-158 °C, 156 - 158 °C | |
Record name | Aminolevulinic acid | |
Source | DrugBank | |
URL | https://www.drugbank.ca/drugs/DB00855 | |
Description | The DrugBank database is a unique bioinformatics and cheminformatics resource that combines detailed drug (i.e. chemical, pharmacological and pharmaceutical) data with comprehensive drug target (i.e. sequence, structure, and pathway) information. | |
Explanation | Creative Common's Attribution-NonCommercial 4.0 International License (http://creativecommons.org/licenses/by-nc/4.0/legalcode) | |
Record name | 5-Aminolevulinic acid | |
Source | Human Metabolome Database (HMDB) | |
URL | http://www.hmdb.ca/metabolites/HMDB0001149 | |
Description | The Human Metabolome Database (HMDB) is a freely available electronic database containing detailed information about small molecule metabolites found in the human body. | |
Explanation | HMDB is offered to the public as a freely available resource. Use and re-distribution of the data, in whole or in part, for commercial purposes requires explicit permission of the authors and explicit acknowledgment of the source material (HMDB) and the original publication (see the HMDB citing page). We ask that users who download significant portions of the database cite the HMDB paper in any resulting publications. | |
Retrosynthesis Analysis
AI-Powered Synthesis Planning: Our tool employs the Template_relevance Pistachio, Template_relevance Bkms_metabolic, Template_relevance Pistachio_ringbreaker, Template_relevance Reaxys, Template_relevance Reaxys_biocatalysis model, leveraging a vast database of chemical reactions to predict feasible synthetic routes.
One-Step Synthesis Focus: Specifically designed for one-step synthesis, it provides concise and direct routes for your target compounds, streamlining the synthesis process.
Accurate Predictions: Utilizing the extensive PISTACHIO, BKMS_METABOLIC, PISTACHIO_RINGBREAKER, REAXYS, REAXYS_BIOCATALYSIS database, our tool offers high-accuracy predictions, reflecting the latest in chemical research and data.
Strategy Settings
Precursor scoring | Relevance Heuristic |
---|---|
Min. plausibility | 0.01 |
Model | Template_relevance |
Template Set | Pistachio/Bkms_metabolic/Pistachio_ringbreaker/Reaxys/Reaxys_biocatalysis |
Top-N result to add to graph | 6 |
Feasible Synthetic Routes
Disclaimer and Information on In-Vitro Research Products
Please be aware that all articles and product information presented on BenchChem are intended solely for informational purposes. The products available for purchase on BenchChem are specifically designed for in-vitro studies, which are conducted outside of living organisms. In-vitro studies, derived from the Latin term "in glass," involve experiments performed in controlled laboratory settings using cells or tissues. It is important to note that these products are not categorized as medicines or drugs, and they have not received approval from the FDA for the prevention, treatment, or cure of any medical condition, ailment, or disease. We must emphasize that any form of bodily introduction of these products into humans or animals is strictly prohibited by law. It is essential to adhere to these guidelines to ensure compliance with legal and ethical standards in research and experimentation.