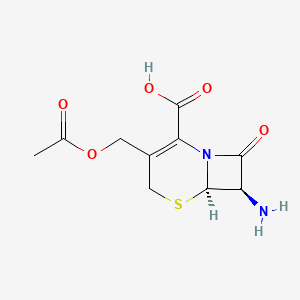
7-Aminocephalosporanic acid
Overview
Description
7-Aminocephalosporanic acid is a core chemical structure used in the synthesis of cephalosporin antibiotics. It is derived from cephalosporin C, which is produced by the fungus Acremonium chrysogenum. Cephalosporins are a large group of β-lactam antibiotics that are closely related to penicillins and are used to treat a variety of bacterial infections .
Mechanism of Action
Target of Action
7-Aminocephalosporanic acid (7-ACA) is a core compound used in the production of semi-synthetic cephalosporins, a class of β-lactam antibiotics . The primary targets of 7-ACA, like other β-lactam antibiotics, are the penicillin-binding proteins (PBPs) located inside the bacterial cell wall . These proteins are essential for the synthesis of the bacterial cell wall, and their inhibition leads to the disruption of cell wall construction .
Mode of Action
7-ACA, as a β-lactam antibiotic, works by mimicking the D-Ala-D-Ala terminal of the peptide chain of the bacterial cell wall. It binds to the active site of PBPs, inhibiting the transpeptidation step of peptidoglycan synthesis during bacterial cell wall biosynthesis . This inhibition disrupts the bacterial cell wall construction, leading to cell lysis and death .
Biochemical Pathways
The synthesis of 7-ACA involves the transformation of cephalosporin C (CPC), a natural secondary metabolite produced by the filamentous fungus Acremonium chrysogenum . This transformation can occur through a two-step or one-step enzymatic conversion process . The first enzyme modifies the 7-aminoadipyl side chain of CPC to generate glutaryl-7-aminocephalosporanic acid (GL-7-ACA). The second enzyme, GL-7-ACA acylase, converts GL-7-ACA to the final product, 7-ACA .
Pharmacokinetics
The pharmacokinetics of 7-ACA and its derivatives, the cephalosporins, vary widely. Factors such as lipophilicity and the presence of sulfur or oxygen atoms at specific positions can significantly affect the drug’s absorption, distribution, metabolism, and excretion (ADME) properties . .
Result of Action
The result of 7-ACA’s action is the inhibition of bacterial growth. By disrupting the synthesis of the peptidoglycan layer of the bacterial cell wall, 7-ACA causes bacterial cell lysis and death . This makes 7-ACA and its derivatives effective in treating and preventing bacterial diseases caused by both gram-positive and gram-negative bacteria .
Action Environment
The action of 7-ACA can be influenced by various environmental factors. For instance, the pH of the environment can affect the predominant form of 7-ACA. At pH below 2.02, the predominant form of 7-ACA is cationic, while at pH above 4.42, it is anionic . Additionally, the production of 7-ACA from CPC can be influenced by factors such as incubation times, temperatures, and pH values .
Biochemical Analysis
Biochemical Properties
7-ACA interacts with various enzymes and proteins. It contains a dihydro-thiazine ring and a β-lactam ring, which have a resistant effect on the class of Staphylococcus aureus penicillinase . The modifications of 7-ACA side-chains can lead to the alteration of pharmacokinetic properties and receptor binding affinity, thus creating a new class of cephalosporin antibiotics with important clinical uses .
Cellular Effects
7-ACA has significant effects on various types of cells and cellular processes. It is widely used for treating and preventing bacterial diseases by disrupting the synthesis of the peptidoglycan layer of cell walls in both gram-positive and gram-negative bacteria . The modifications of 7-ACA side-chains can affect the antibacterial activity and lead to alterations in cell function .
Molecular Mechanism
The mechanism of action of 7-ACA is primarily through its interactions with biomolecules. It exerts its effects at the molecular level, including binding interactions with biomolecules, enzyme inhibition or activation, and changes in gene expression . The modifications of the 7-ACA nucleus and substitutions on the side chains via semisynthetic means have produced differences among cephalosporins in antibacterial spectra, beta-lactamase sensitivities, and pharmacokinetics .
Temporal Effects in Laboratory Settings
Over time, the effects of 7-ACA can change in laboratory settings. Information on the product’s stability, degradation, and any long-term effects on cellular function observed in in vitro or in vivo studies is crucial. The production level of 7-ACA is nearly equivalent to the total β-lactams biosynthesized by the parental overproducing strain .
Metabolic Pathways
7-ACA is involved in the metabolic pathways of cephalosporin antibiotics. It is usually produced from cephalosporin-C (CPC) by the enzyme cephalosporin acylases . These enzymes are the groups of β-lactam acylases, highly specialized peptidases that are capable of cleaving the amide bond between a β-lactam nucleus and a side chain without damaging the β-lactam ring .
Subcellular Localization
Given that 7-ACA is a key intermediate in the production of semisynthetic cephalosporins, it is likely involved in cellular processes in specific compartments or organelles related to antibiotic synthesis .
Preparation Methods
Synthetic Routes and Reaction Conditions: 7-Aminocephalosporanic acid can be obtained through chemoenzymatic hydrolysis of cephalosporin C. This process involves the use of cephalosporin acylase enzymes, which cleave the amide bond between the β-lactam nucleus and the side chain without damaging the β-lactam ring .
Industrial Production Methods: The industrial production of this compound typically involves the fermentation of Acremonium chrysogenum to produce cephalosporin C, followed by enzymatic conversion to this compound. This process can be carried out in a single step or two-step enzymatic conversion .
Chemical Reactions Analysis
Types of Reactions: 7-Aminocephalosporanic acid undergoes various chemical reactions, including acylation, oxidation, and substitution. These reactions are essential for the synthesis of different cephalosporin antibiotics .
Common Reagents and Conditions:
Acylation: This reaction involves the use of acylating agents such as benzoyl chloride and cinnamoyl chloride.
Oxidation: Oxidizing agents like m-chloroperbenzoic acid can be used to oxidize this compound.
Substitution: Various substituents can be introduced at the C3 and C7 positions of the β-lactam nucleus to create different cephalosporin derivatives.
Major Products: The major products formed from these reactions are different cephalosporin antibiotics, such as cefotaxime and cefpodoxime proxetil .
Scientific Research Applications
7-Aminocephalosporanic acid is widely used in the pharmaceutical industry for the production of semi-synthetic cephalosporins. These antibiotics are crucial for treating bacterial infections, especially those caused by resistant strains . Additionally, this compound derivatives have shown potential bioactivities, including enzyme inhibition .
Comparison with Similar Compounds
6-Aminopenicillanic acid: This compound is the core structure for penicillin antibiotics.
7-Aminodeacetoxycephalosporanic acid: Another core structure used in the synthesis of cephalosporin antibiotics.
Uniqueness: 7-Aminocephalosporanic acid is unique due to its ability to form a wide variety of cephalosporin antibiotics with different spectra of activity and resistance profiles. Its β-lactam ring structure allows for modifications at the C3 and C7 positions, leading to the development of antibiotics with enhanced properties .
Properties
IUPAC Name |
(6R,7R)-3-(acetyloxymethyl)-7-amino-8-oxo-5-thia-1-azabicyclo[4.2.0]oct-2-ene-2-carboxylic acid | |
---|---|---|
Source | PubChem | |
URL | https://pubchem.ncbi.nlm.nih.gov | |
Description | Data deposited in or computed by PubChem | |
InChI |
InChI=1S/C10H12N2O5S/c1-4(13)17-2-5-3-18-9-6(11)8(14)12(9)7(5)10(15)16/h6,9H,2-3,11H2,1H3,(H,15,16)/t6-,9-/m1/s1 | |
Source | PubChem | |
URL | https://pubchem.ncbi.nlm.nih.gov | |
Description | Data deposited in or computed by PubChem | |
InChI Key |
HSHGZXNAXBPPDL-HZGVNTEJSA-N | |
Source | PubChem | |
URL | https://pubchem.ncbi.nlm.nih.gov | |
Description | Data deposited in or computed by PubChem | |
Canonical SMILES |
CC(=O)OCC1=C(N2C(C(C2=O)N)SC1)C(=O)O | |
Source | PubChem | |
URL | https://pubchem.ncbi.nlm.nih.gov | |
Description | Data deposited in or computed by PubChem | |
Isomeric SMILES |
CC(=O)OCC1=C(N2[C@@H]([C@@H](C2=O)N)SC1)C(=O)O | |
Source | PubChem | |
URL | https://pubchem.ncbi.nlm.nih.gov | |
Description | Data deposited in or computed by PubChem | |
Molecular Formula |
C10H12N2O5S | |
Source | PubChem | |
URL | https://pubchem.ncbi.nlm.nih.gov | |
Description | Data deposited in or computed by PubChem | |
DSSTOX Substance ID |
DTXSID9045342 | |
Record name | 7-Aminocephalosporanic acid | |
Source | EPA DSSTox | |
URL | https://comptox.epa.gov/dashboard/DTXSID9045342 | |
Description | DSSTox provides a high quality public chemistry resource for supporting improved predictive toxicology. | |
Molecular Weight |
272.28 g/mol | |
Source | PubChem | |
URL | https://pubchem.ncbi.nlm.nih.gov | |
Description | Data deposited in or computed by PubChem | |
CAS No. |
957-68-6 | |
Record name | 7-Aminocephalosporanic acid | |
Source | CAS Common Chemistry | |
URL | https://commonchemistry.cas.org/detail?cas_rn=957-68-6 | |
Description | CAS Common Chemistry is an open community resource for accessing chemical information. Nearly 500,000 chemical substances from CAS REGISTRY cover areas of community interest, including common and frequently regulated chemicals, and those relevant to high school and undergraduate chemistry classes. This chemical information, curated by our expert scientists, is provided in alignment with our mission as a division of the American Chemical Society. | |
Explanation | The data from CAS Common Chemistry is provided under a CC-BY-NC 4.0 license, unless otherwise stated. | |
Record name | 7-Aminocephalosporanic acid | |
Source | ChemIDplus | |
URL | https://pubchem.ncbi.nlm.nih.gov/substance/?source=chemidplus&sourceid=0000957686 | |
Description | ChemIDplus is a free, web search system that provides access to the structure and nomenclature authority files used for the identification of chemical substances cited in National Library of Medicine (NLM) databases, including the TOXNET system. | |
Record name | 7-Aminocephalosporanic acid | |
Source | EPA DSSTox | |
URL | https://comptox.epa.gov/dashboard/DTXSID9045342 | |
Description | DSSTox provides a high quality public chemistry resource for supporting improved predictive toxicology. | |
Record name | 3-acetoxymethylen-7-amino-8-oxo-5-thia-1-azabicyclo[4.2.0]oct-2-ene-2-carboxylic acid | |
Source | European Chemicals Agency (ECHA) | |
URL | https://echa.europa.eu/substance-information/-/substanceinfo/100.012.259 | |
Description | The European Chemicals Agency (ECHA) is an agency of the European Union which is the driving force among regulatory authorities in implementing the EU's groundbreaking chemicals legislation for the benefit of human health and the environment as well as for innovation and competitiveness. | |
Explanation | Use of the information, documents and data from the ECHA website is subject to the terms and conditions of this Legal Notice, and subject to other binding limitations provided for under applicable law, the information, documents and data made available on the ECHA website may be reproduced, distributed and/or used, totally or in part, for non-commercial purposes provided that ECHA is acknowledged as the source: "Source: European Chemicals Agency, http://echa.europa.eu/". Such acknowledgement must be included in each copy of the material. ECHA permits and encourages organisations and individuals to create links to the ECHA website under the following cumulative conditions: Links can only be made to webpages that provide a link to the Legal Notice page. | |
Record name | 7-AMINOCEPHALOSPORANIC ACID | |
Source | FDA Global Substance Registration System (GSRS) | |
URL | https://gsrs.ncats.nih.gov/ginas/app/beta/substances/9XI67897RG | |
Description | The FDA Global Substance Registration System (GSRS) enables the efficient and accurate exchange of information on what substances are in regulated products. Instead of relying on names, which vary across regulatory domains, countries, and regions, the GSRS knowledge base makes it possible for substances to be defined by standardized, scientific descriptions. | |
Explanation | Unless otherwise noted, the contents of the FDA website (www.fda.gov), both text and graphics, are not copyrighted. They are in the public domain and may be republished, reprinted and otherwise used freely by anyone without the need to obtain permission from FDA. Credit to the U.S. Food and Drug Administration as the source is appreciated but not required. | |
Retrosynthesis Analysis
AI-Powered Synthesis Planning: Our tool employs the Template_relevance Pistachio, Template_relevance Bkms_metabolic, Template_relevance Pistachio_ringbreaker, Template_relevance Reaxys, Template_relevance Reaxys_biocatalysis model, leveraging a vast database of chemical reactions to predict feasible synthetic routes.
One-Step Synthesis Focus: Specifically designed for one-step synthesis, it provides concise and direct routes for your target compounds, streamlining the synthesis process.
Accurate Predictions: Utilizing the extensive PISTACHIO, BKMS_METABOLIC, PISTACHIO_RINGBREAKER, REAXYS, REAXYS_BIOCATALYSIS database, our tool offers high-accuracy predictions, reflecting the latest in chemical research and data.
Strategy Settings
Precursor scoring | Relevance Heuristic |
---|---|
Min. plausibility | 0.01 |
Model | Template_relevance |
Template Set | Pistachio/Bkms_metabolic/Pistachio_ringbreaker/Reaxys/Reaxys_biocatalysis |
Top-N result to add to graph | 6 |
Feasible Synthetic Routes
Q1: What are the advantages of using enzymes in 7-ACA production?
A1: Enzymatic processes offer several advantages over chemical methods, including milder reaction conditions, higher yields, and reduced environmental impact. [, ] For instance, using immobilized DAAO and GLA in a two-step process led to a 72% overall yield of 7-ACA from deacetyl cephalosporin C (DCPC). []
Q2: Are there efforts to further improve the enzymatic production of 7-ACA?
A2: Yes, researchers are actively exploring one-pot enzymatic processes using various enzyme combinations to simplify production and reduce costs. [] These approaches include using cephalosporin C acylase, modified forms of GLA, and combinations of DAAO and GLA, with or without catalase. []
Q3: Can γ-glutamyltranspeptidase (GGT) be used to produce 7-ACA?
A3: While not traditionally used, research has shown that GGT from Bacillus subtilis possesses inherent glutaryl-7-aminocephalosporanic acid acylase activity. [] This activity can be significantly enhanced through protein engineering, potentially offering an alternative route to 7-ACA production. []
Q4: How can the activity of enzymes involved in 7-ACA production be enhanced?
A4: Protein engineering techniques, such as site-directed mutagenesis and directed evolution, are employed to enhance enzyme activity, stability, and substrate specificity. [, ] For example, modifying amino acid residues in GGT that interact with γ-glutamyl compounds or are located in the active center's peripheral region can increase its GL-7-ACA acylase activity. []
Q5: What is the role of immobilization in the enzymatic production of 7-ACA?
A5: Enzyme immobilization offers benefits like enhanced stability, reusability, and ease of separation from the reaction mixture. [, , ] Immobilized DAAO and GLA have demonstrated extended operational stability in the production of 7-ACA. [, ]
Q6: What is the molecular formula and weight of 7-ACA?
A6: The molecular formula of 7-ACA is C8H10N2O3S, and its molecular weight is 214.24 g/mol.
Q7: Is there a spectroscopic method to quantify 7-ACA?
A7: Yes, a colorimetric method has been developed for the determination of 7-ACA and related compounds. [] This method offers a reproducible way to quantify 7-ACA.
Q8: What is the agglomeration phenomenon of 7-ACA crystals, and how does it relate to the crystallization process?
A8: 7-ACA crystals produced using the acid method tend to agglomerate at pH values higher than 1.5, while those produced using the basic method do not. [] This difference is attributed to variations in surface characteristics, such as fractal dimension and wettability, resulting from the different crystallization processes. []
Q9: How does the pH of the solution affect the extraction of 7-ACA?
A9: The extraction of 7-ACA is influenced by the pH of the solution and the type of carrier used. [] Ion-pair extraction with secondary and tertiary amines shows a decrease in distribution coefficient with increasing pH, while ion exchange extraction with quaternary ammonium salts displays the opposite trend. []
Q10: How do cephalosporins, derived from 7-ACA, exert their antibacterial activity?
A10: Cephalosporins, like penicillins, function by inhibiting bacterial cell wall synthesis. [] They interfere with the transpeptidation reaction, preventing the cross-linking of peptidoglycans, essential components of the bacterial cell wall.
Q11: What are the advantages of semisynthetic cephalosporins over naturally occurring ones?
A11: Semisynthetic cephalosporins, derived from 7-ACA, often possess improved pharmacological properties compared to their natural counterparts. [, ] These enhancements may include a broader spectrum of activity, increased potency, improved pharmacokinetic properties, and enhanced stability against bacterial enzymes like beta-lactamases.
Q12: What are activated esters of 7-ACA, and why are they important?
A12: Activated esters of 7-ACA are derivatives designed to react readily with other molecules, enabling the synthesis of a wide array of cephalosporin antibiotics. [] These activated esters provide alternative and potentially more efficient routes to producing existing and novel cephalosporins. []
Q13: What are the challenges associated with the use of 7-ACA derivatives as antibiotics?
A13: The primary challenge is the emergence and spread of bacterial resistance to cephalosporin antibiotics. [, , ] This resistance can arise through various mechanisms, such as the production of β-lactamases, which inactivate cephalosporins by hydrolyzing their β-lactam ring. []
Q14: Is there any research on mitigating the resistance to 7-ACA derived antibiotics?
A14: Yes, research focuses on developing new cephalosporin analogs with improved stability against β-lactamases and exploring combination therapies with β-lactamase inhibitors. [, ]
Q15: What is the future direction of research on 7-ACA?
A15: Future research will likely focus on developing more efficient and sustainable production methods for 7-ACA, engineering enzymes with enhanced catalytic properties, and designing novel cephalosporin analogs with improved activity and reduced susceptibility to resistance mechanisms. [, , ]
Disclaimer and Information on In-Vitro Research Products
Please be aware that all articles and product information presented on BenchChem are intended solely for informational purposes. The products available for purchase on BenchChem are specifically designed for in-vitro studies, which are conducted outside of living organisms. In-vitro studies, derived from the Latin term "in glass," involve experiments performed in controlled laboratory settings using cells or tissues. It is important to note that these products are not categorized as medicines or drugs, and they have not received approval from the FDA for the prevention, treatment, or cure of any medical condition, ailment, or disease. We must emphasize that any form of bodily introduction of these products into humans or animals is strictly prohibited by law. It is essential to adhere to these guidelines to ensure compliance with legal and ethical standards in research and experimentation.