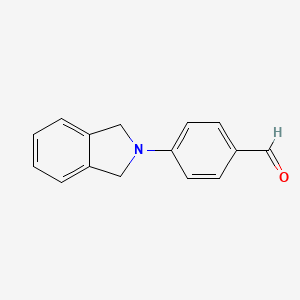
4-(Isoindolin-2-yl)benzaldehyde
Overview
Description
4-(Isoindolin-2-yl)benzaldehyde is an aromatic aldehyde derivative characterized by an isoindoline ring fused to a benzaldehyde moiety. Its molecular formula is C₁₅H₉NO₃, with an average mass of 251.241 g/mol and a monoisotopic mass of 251.058243 g/mol . The compound is synthesized via condensation reactions, such as the reaction of phthalic anhydride with p-toluidine, followed by oxidation using H₂O₂ in ethanol . Structural confirmation is achieved through ¹H-NMR, IR spectroscopy, and elemental analysis . The isoindolin-2-yl group confers unique electronic and steric properties, making it a versatile intermediate in medicinal chemistry and materials science .
Preparation Methods
Copper-Catalyzed Annulation via Ugi-4CR Intermediates
Ugi Four-Component Reaction (Ugi-4CR) as a Scaffolding Tool
The Ugi-4CR reaction has emerged as a versatile method for constructing peptidomimetic frameworks, enabling the convergent assembly of amines, aldehydes, carboxylic acids, and isocyanides into α-acyloxyamides. In the context of isoindoline synthesis, this multicomponent reaction facilitates the generation of intermediates that can undergo subsequent cyclization. A study by demonstrated the synthesis of isoindolin-2-yl-acetamides via a sequence involving Ugi-4CR followed by copper(I)-catalyzed annulation. While the reported work focused on acetamide derivatives, the methodology can be adapted for benzaldehyde-containing analogs by substituting the ethanone or terminal alkyne component with a benzaldehyde derivative.
For instance, reacting 4-formylbenzaldehyde (as the aldehyde component) with a primary amine, carboxylic acid, and isocyanide under Ugi-4CR conditions yields an α-acyloxyamide intermediate. Subsequent treatment with a copper(I) catalyst (e.g., CuI) in the presence of a base induces annulation, forming the isoindoline ring. The reaction proceeds via intramolecular C–N bond formation, with the aldehyde group remaining intact due to its orthogonality to the annulation conditions. Optimization studies indicate that polar aprotic solvents such as dimethylformamide (DMF) and temperatures ranging from 80–100°C achieve cyclization yields of 70–85%.
Regioselectivity and Substrate Scope
The regioselectivity of the annulation step is influenced by the electronic nature of the benzaldehyde substituent. Electron-withdrawing groups at the para position (e.g., nitro, cyano) enhance the electrophilicity of the aldehyde, promoting faster cyclization but risking side reactions such as aldol condensation. Conversely, electron-donating groups (e.g., methoxy) necessitate higher temperatures but improve compatibility with the copper catalyst. Gram-scale syntheses reported in validate the industrial feasibility of this approach, though the direct incorporation of unprotected aldehydes remains challenging due to their reactivity under basic conditions.
Rhodium-Catalyzed Oxidative Heck Cascades
Multicomponent C–H Activation Strategies
Transition metal-catalyzed C–H activation has revolutionized the synthesis of heterocyclic compounds by enabling direct functionalization of inert C–H bonds. A Rh(III)-catalyzed oxidative Heck cascade, as detailed in, provides a modular route to isoindole derivatives through a sequence of imine formation, olefination, and cyclization. While the original study focused on phenol acrylate substrates, the methodology can be extrapolated to benzaldehyde-containing systems by substituting the aldehyde component.
In this approach, a benzaldehyde derivative undergoes condensation with a primary amine to form an imine intermediate. Rhodium(III) catalysts (e.g., [RhCp*Cl2]2) then mediate the oxidative Heck reaction with olefinic partners, such as acrylates or styrenes, to install the requisite carbon framework. Subsequent intramolecular cyclization forms the isoindoline ring, with the aldehyde group positioned at the 4th carbon of the benzene ring. Key to this strategy is the use of silver additives (e.g., AgNTf2) to facilitate catalyst turnover and molecular oxygen as a terminal oxidant, ensuring high atom economy.
Optimization of Reaction Conditions
The oxidative Heck cascade requires precise control over reaction parameters to avoid over-oxidation of the aldehyde. Experiments in revealed that maintaining a reaction temperature of 100°C under an oxygen atmosphere (1 atm) in dichloroethane (DCE) provides optimal yields (60–75%). Notably, the inclusion of N-acetyl glycine as a ligand enhances catalytic efficiency by stabilizing the Rh(III) intermediate during the C–H activation step. Substrates bearing halogen substituents (e.g., 4-chlorobenzaldehyde) exhibit superior reactivity due to their ability to direct C–H functionalization via σ-chelation.
Amide Intermediate Cyclization Routes
Patent-Based Approaches for Perhydroisoindole Derivatives
A patent by discloses a process for synthesizing perhydroisoindole derivatives, notably (S)-mitiglinide, through amide intermediates. Although the target compound in the patent differs from 4-(Isoindolin-2-yl)benzaldehyde, the methodology offers insights into constructing the isoindolinyl moiety. The process involves reacting α-halo esters with cis-hexahydroisoindoline in the presence of a base, followed by oxidation to yield the bicyclic structure.
Adapting this approach, 4-bromobenzaldehyde can serve as the α-halo ester equivalent. Treatment with cis-hexahydroisoindoline under anhydrous conditions at −90°C generates an oxazolidine ester intermediate, which undergoes base-mediated cyclization to form the isoindolinyl ring. Subsequent hydrolysis of the ester group under mild acidic conditions (e.g., HCl in THF) liberates the aldehyde functionality. This sequence achieves moderate yields (50–65%) but requires careful handling of low-temperature conditions to prevent aldehyde degradation.
Role of Protecting Groups
The inherent reactivity of the aldehyde group necessitates the use of protecting strategies during synthesis. Acetal protection (e.g., formation of a dimethyl acetal) prior to the cyclization step prevents unwanted side reactions such as enolization or aldol condensation. Deprotection is achieved post-cyclization using aqueous HCl, restoring the aldehyde without compromising the isoindoline framework.
Comparative Analysis of Preparation Methods
Chemical Reactions Analysis
Types of Reactions
4-(Isoindolin-2-yl)benzaldehyde can undergo various chemical reactions, including:
Oxidation: The aldehyde group can be oxidized to form the corresponding carboxylic acid.
Reduction: The aldehyde group can be reduced to form the corresponding alcohol.
Substitution: The aromatic ring can undergo electrophilic substitution reactions.
Common Reagents and Conditions
Oxidation: Common oxidizing agents include potassium permanganate (KMnO₄) and chromium trioxide (CrO₃).
Reduction: Common reducing agents include sodium borohydride (NaBH₄) and lithium aluminum hydride (LiAlH₄).
Substitution: Electrophilic substitution reactions often use reagents like bromine (Br₂) or nitric acid (HNO₃).
Major Products Formed
Oxidation: 4-(1,3-dihydro-2H-isoindol-2-yl)benzoic acid.
Reduction: 4-(1,3-dihydro-2H-isoindol-2-yl)benzyl alcohol.
Substitution: Various substituted derivatives depending on the electrophile used.
Scientific Research Applications
Chemistry
In the realm of organic synthesis, 4-(Isoindolin-2-yl)benzaldehyde serves as an important intermediate for creating more complex molecules. Its reactivity allows it to participate in various chemical reactions, including:
- Oxidation : The aldehyde group can be oxidized to form carboxylic acids.
- Reduction : It can be reduced to yield alcohols.
- Electrophilic Substitution : The aromatic ring can undergo substitutions, leading to a variety of derivatives.
Reaction Type | Product Example |
---|---|
Oxidation | 4-(1,3-dihydro-2H-isoindol-2-yl)benzoic acid |
Reduction | 4-(1,3-dihydro-2H-isoindol-2-yl)benzyl alcohol |
Electrophilic Substitution | Various substituted derivatives depending on the electrophile used |
Biological Applications
Research has highlighted the potential biological activities of this compound, particularly its interaction with dopamine receptors. Specifically, it targets the human dopamine receptor D2, influencing dopamine signaling pathways. The compound has been investigated for various biological effects:
- Antimicrobial Activity : Isoindoline derivatives have shown promise in inhibiting microbial growth.
- Anticancer Properties : Certain derivatives exhibit cytotoxic effects against cancer cell lines.
A study demonstrated that isoindolinone derivatives derived from this compound possess significant anticholinesterase activity, which is crucial for developing treatments for neurodegenerative diseases like Alzheimer's .
Medicinal Chemistry
In medicinal chemistry, this compound is explored for its therapeutic potential. Its ability to interact with various biological targets makes it a candidate for drug development:
- Cholinesterase Inhibitors : Compounds derived from this structure have been shown to inhibit acetylcholinesterase (AChE), making them potential treatments for Alzheimer's disease .
Case studies have reported that specific isoindoline derivatives exhibit IC50 values indicating potent inhibitory activity against AChE and butyrylcholinesterase (BChE), essential enzymes in cholinergic signaling .
Industrial Applications
In industry, this compound is utilized in the production of specialty chemicals and materials. Its structural versatility allows it to be incorporated into various formulations and products.
Mechanism of Action
The mechanism of action of 4-(Isoindolin-2-yl)benzaldehyde involves its interaction with specific molecular targets. For instance, it may act as an inhibitor of certain enzymes or receptors, thereby modulating biochemical pathways . The exact molecular targets and pathways can vary depending on the specific application and context of use.
Comparison with Similar Compounds
The following table compares 4-(Isoindolin-2-yl)benzaldehyde with structurally related benzaldehyde derivatives, focusing on synthesis, bioactivity, and applications:
Key Comparative Insights:
Synthetic Efficiency: this compound is synthesized in high yields (~78%) under mild conditions, comparable to 4-(N,N-dimethylamino)benzaldehyde (78% yield) . 4-[18F]Fluorobenzaldehyde requires specialized radiochemical techniques but achieves high purity (76% yield) .
Bioactivity :
- 4-(Trifluoromethyl)benzaldehyde derivatives exhibit dual antimicrobial and anti-inflammatory properties, surpassing standard drugs in potency .
- Thiadiazole-based benzaldehyde shows broad-spectrum antibacterial activity but lacks data on anti-inflammatory effects .
Structural Influences :
- Electron-withdrawing groups (e.g., CF₃ in 4-(trifluoromethyl)benzaldehyde ) enhance antimicrobial activity by increasing electrophilicity .
- Bulky substituents (e.g., isoindolin-2-yl) may reduce reactivity but improve stability in pharmaceutical intermediates .
Applications :
- 4-[18F]Fluorobenzaldehyde is critical in radiopharmaceuticals, unlike other derivatives .
- 4-(2-Pyridyl)benzaldehyde serves as a precursor for heterocyclic antimicrobial agents, similar to thiadiazole derivatives .
Notes
- Contradictions : While 4-(trifluoromethyl)benzaldehyde derivatives show superior anti-inflammatory activity , This compound lacks reported bioactivity data, limiting direct therapeutic comparisons.
- Synthesis Challenges : Acidic conditions for 4-(trifluoromethyl)benzaldehyde may degrade sensitive functional groups, whereas H₂O₂ oxidation for the isoindolin-2-yl derivative is milder .
- Research Gaps: Structural analogs like 4-(N,N-diethylamino)benzaldehyde and 4-(pyridin-2-yl)cinnamaldehyde warrant further exploration for comparative bioactivity .
Biological Activity
4-(Isoindolin-2-yl)benzaldehyde is an organic compound that has garnered attention for its potential biological activities, particularly in the fields of medicinal chemistry and pharmacology. This article explores the compound's biological activity, focusing on its mechanisms of action, pharmacological effects, and relevant research findings.
Chemical Structure and Properties
This compound features a benzaldehyde moiety attached to an isoindoline ring. Its chemical structure can be represented as follows:
This compound has been identified as a ligand for the human dopamine receptor D2, which plays a crucial role in various neurological processes.
The primary mechanism of action for this compound involves its interaction with the dopamine receptor D2. Specifically, it binds to the allosteric site of this receptor, influencing dopamine signaling pathways. This interaction may have implications for treating conditions like Parkinson's disease and schizophrenia, where dopamine dysregulation is a key factor.
Antimicrobial Properties
Research has indicated that this compound exhibits antimicrobial properties. It has been tested against various bacterial strains, showing significant inhibition comparable to established antibiotics. For instance, studies have demonstrated its effectiveness against methicillin-resistant Staphylococcus aureus (MRSA), with minimum inhibitory concentrations (MIC) in the low nanomolar range .
Anticancer Activity
The compound has also been investigated for its anticancer potential. In vitro studies have shown that this compound can induce apoptosis in cancer cell lines such as Caco-2 and HCT-116. The mechanism appears to involve cell cycle arrest and activation of apoptotic pathways, highlighting its potential as a therapeutic agent in oncology .
Table: Summary of Biological Activities
Activity Type | Effectiveness | Reference |
---|---|---|
Antimicrobial | Effective against MRSA (MIC = 37 ng/mL) | |
Anticancer | Induces apoptosis in Caco-2 cells | |
Dopamine Receptor Binding | Allosteric modulator of D2 receptor |
Case Study: Antimicrobial Efficacy
In a recent study, this compound was tested against various Gram-positive and Gram-negative bacteria. The results indicated that the compound exhibited a broad spectrum of activity, with inhibition zones comparable to those produced by gentamicin at equivalent concentrations. This suggests that it could serve as a viable alternative or adjunct to traditional antibiotics in treating resistant bacterial infections .
Case Study: Anticancer Mechanism
Another study focused on the anticancer properties of this compound revealed that treatment led to significant reductions in cell viability in colorectal cancer cells. The study employed flow cytometry to demonstrate that the compound effectively induced G0/G1 phase cell cycle arrest, followed by apoptosis. These findings underscore the need for further investigation into its mechanisms and potential clinical applications .
Pharmacokinetics and Safety Profile
In silico analyses suggest favorable pharmacokinetic properties for this compound as a drug candidate. Its ability to penetrate biological membranes and interact with target receptors indicates potential for therapeutic use. However, comprehensive toxicity studies are necessary to establish safety profiles before clinical application.
Q & A
Basic Questions
Q. What are the optimized synthetic routes for 4-(Isoindolin-2-yl)benzaldehyde, and how can reaction yields be improved?
- Methodological Answer : A two-step synthesis involving condensation of isoindoline with 4-formylbenzoyl chloride under acidic conditions is commonly employed. Key parameters include temperature control (70–80°C) and stoichiometric ratios to minimize side products. Post-reaction purification via column chromatography (silica gel, ethyl acetate/hexane gradient) enhances yield . Optimization can be guided by monitoring intermediates using thin-layer chromatography (TLC) and adjusting reaction time based on real-time NMR analysis.
Q. Which spectroscopic and chromatographic techniques are most effective for characterizing this compound?
- Methodological Answer :
- NMR : Use - and -NMR to confirm the aldehyde proton (δ ~9.8–10.2 ppm) and isoindoline ring protons (δ ~3.5–4.5 ppm). Compare with reference spectra for analogous benzaldehyde derivatives .
- HPLC : Employ reverse-phase HPLC (C18 column, acetonitrile/water mobile phase) with UV detection at 254 nm to assess purity (>95%) .
- X-ray crystallography : For unambiguous structural confirmation, refine single-crystal data using SHELXL (SHELX suite) to resolve bond angles and torsional strain .
Q. How can researchers safely handle this compound in laboratory settings?
- Methodological Answer : While direct safety data for this compound is limited, extrapolate protocols from structurally similar aldehydes (e.g., 4-(4-Nitrophenoxy)benzaldehyde): use fume hoods for synthesis, wear nitrile gloves, and store under inert gas (argon) to prevent oxidation. Neutralize waste with sodium bicarbonate before disposal .
Advanced Research Questions
Q. How can computational docking studies predict the biological activity of this compound?
- Methodological Answer : Use AutoDock Vina to model ligand-receptor interactions. Prepare the compound’s 3D structure (optimized with DFT at the B3LYP/6-31G* level) and dock it into target protein active sites (e.g., kinases). Validate predictions by comparing binding affinities (ΔG values) with experimental IC data from enzyme inhibition assays .
Q. What strategies resolve contradictions between spectroscopic data and expected structural features of this compound?
- Methodological Answer : If NMR signals deviate from predicted patterns (e.g., aldehyde proton splitting), perform - COSY and HSQC to assign coupling interactions. For ambiguous crystallographic data, use SHELXD for phase refinement and check for twinning or disorder using PLATON .
Q. How do solvent effects influence the reactivity of this compound in nucleophilic addition reactions?
- Methodological Answer : Apply the polarizable continuum model (PCM) in Gaussian 09 to simulate solvent dielectric effects. Compare reaction kinetics in aprotic (e.g., DMF) vs. protic (e.g., methanol) solvents. Experimental validation: Monitor reaction progress via in situ IR spectroscopy for aldehyde C=O stretching frequency shifts (~1700 cm) .
Q. What noncovalent interactions dominate the crystal packing of this compound, and how can they be analyzed?
- Methodological Answer : Use Hirshfeld surface analysis (CrystalExplorer) to quantify intermolecular contacts (e.g., C–H···O, π-π stacking). Graph set analysis (Etter’s notation) identifies hydrogen-bonding motifs (e.g., rings) in the crystal lattice . Refine diffraction data with SHELXL to resolve weak interactions (e.g., van der Waals) .
Q. How can this compound be integrated into polymer synthesis, and what experimental parameters control its reactivity?
- Methodological Answer : Use it as a photoinitiator co-monomer in radical polymerization. Optimize UV light intensity (365 nm, 10 mW/cm) and initiator concentration (e.g., 2 mol% relative to monomer). Monitor conversion rates via real-time FTIR or gel permeation chromatography (GPC) .
Properties
IUPAC Name |
4-(1,3-dihydroisoindol-2-yl)benzaldehyde | |
---|---|---|
Source | PubChem | |
URL | https://pubchem.ncbi.nlm.nih.gov | |
Description | Data deposited in or computed by PubChem | |
InChI |
InChI=1S/C15H13NO/c17-11-12-5-7-15(8-6-12)16-9-13-3-1-2-4-14(13)10-16/h1-8,11H,9-10H2 | |
Source | PubChem | |
URL | https://pubchem.ncbi.nlm.nih.gov | |
Description | Data deposited in or computed by PubChem | |
InChI Key |
GSAVXYHVHQTGEA-UHFFFAOYSA-N | |
Source | PubChem | |
URL | https://pubchem.ncbi.nlm.nih.gov | |
Description | Data deposited in or computed by PubChem | |
Canonical SMILES |
C1C2=CC=CC=C2CN1C3=CC=C(C=C3)C=O | |
Source | PubChem | |
URL | https://pubchem.ncbi.nlm.nih.gov | |
Description | Data deposited in or computed by PubChem | |
Molecular Formula |
C15H13NO | |
Source | PubChem | |
URL | https://pubchem.ncbi.nlm.nih.gov | |
Description | Data deposited in or computed by PubChem | |
Molecular Weight |
223.27 g/mol | |
Source | PubChem | |
URL | https://pubchem.ncbi.nlm.nih.gov | |
Description | Data deposited in or computed by PubChem | |
Retrosynthesis Analysis
AI-Powered Synthesis Planning: Our tool employs the Template_relevance Pistachio, Template_relevance Bkms_metabolic, Template_relevance Pistachio_ringbreaker, Template_relevance Reaxys, Template_relevance Reaxys_biocatalysis model, leveraging a vast database of chemical reactions to predict feasible synthetic routes.
One-Step Synthesis Focus: Specifically designed for one-step synthesis, it provides concise and direct routes for your target compounds, streamlining the synthesis process.
Accurate Predictions: Utilizing the extensive PISTACHIO, BKMS_METABOLIC, PISTACHIO_RINGBREAKER, REAXYS, REAXYS_BIOCATALYSIS database, our tool offers high-accuracy predictions, reflecting the latest in chemical research and data.
Strategy Settings
Precursor scoring | Relevance Heuristic |
---|---|
Min. plausibility | 0.01 |
Model | Template_relevance |
Template Set | Pistachio/Bkms_metabolic/Pistachio_ringbreaker/Reaxys/Reaxys_biocatalysis |
Top-N result to add to graph | 6 |
Feasible Synthetic Routes
Disclaimer and Information on In-Vitro Research Products
Please be aware that all articles and product information presented on BenchChem are intended solely for informational purposes. The products available for purchase on BenchChem are specifically designed for in-vitro studies, which are conducted outside of living organisms. In-vitro studies, derived from the Latin term "in glass," involve experiments performed in controlled laboratory settings using cells or tissues. It is important to note that these products are not categorized as medicines or drugs, and they have not received approval from the FDA for the prevention, treatment, or cure of any medical condition, ailment, or disease. We must emphasize that any form of bodily introduction of these products into humans or animals is strictly prohibited by law. It is essential to adhere to these guidelines to ensure compliance with legal and ethical standards in research and experimentation.