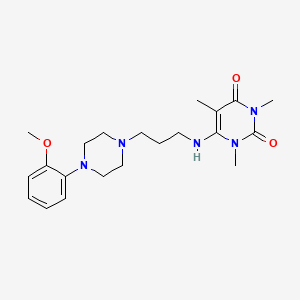
5-Methylurapidil
Overview
Description
5-Methylurapidil is a selective α1-adrenoceptor (α1-AR) antagonist with high affinity for the α1A- and α1D-adrenoceptor subtypes. It is widely used in pharmacological research to dissect the functional roles of α1-AR subtypes in cardiovascular, renal, and smooth muscle systems . Structurally, it belongs to the arylpiperazine class of compounds and is characterized by its competitive antagonism, reversibly inhibiting agonist-induced responses such as vasoconstriction and smooth muscle contraction. Its subtype selectivity (α1A > α1D >> α1B) makes it a critical tool for distinguishing α1-AR-mediated pathways in complex tissues .
Preparation Methods
Synthetic Routes and Reaction Conditions: The preparation of Urapidil-5-methyl involves several steps:
Mixing 1,3-dimethyl-6-semicarbazide pyrimidine and 3-amino-1-propanol: to prepare 6-(3-hydroxypropyl amino)-1,3-dimethyl uracil.
Reacting 6-(3-hydroxypropyl amino)-1,3-dimethyl uracil with thionyl chloride: to prepare 6-(3-chloropropylamino)-1,3-dimethyl uracil.
Reacting 6-(3-chloropropylamino)-1,3-dimethyluracil with 1-(2-methoxyphenyl) piperazine hydrochloride: to obtain Urapidil.
Industrial Production Methods: The industrial production of Urapidil-5-methyl follows similar synthetic routes but is optimized for higher yield and purity. The process involves the use of readily available reagents, minimal side reactions, and efficient purification steps .
Chemical Reactions Analysis
Types of Reactions: Urapidil-5-methyl undergoes various chemical reactions, including:
Oxidation: This reaction involves the addition of oxygen or the removal of hydrogen.
Reduction: This reaction involves the addition of hydrogen or the removal of oxygen.
Substitution: This reaction involves the replacement of one atom or group of atoms with another.
Common Reagents and Conditions:
Oxidation: Common reagents include potassium permanganate and hydrogen peroxide.
Reduction: Common reagents include lithium aluminum hydride and sodium borohydride.
Substitution: Common reagents include halogens and nucleophiles.
Major Products Formed: The major products formed from these reactions depend on the specific reagents and conditions used. For example, oxidation may produce carboxylic acids, reduction may produce alcohols, and substitution may produce halogenated compounds .
Scientific Research Applications
Pharmacological Profile
5-Methylurapidil exhibits a high affinity for alpha-1A adrenoceptors, making it a valuable tool for differentiating between subtypes of alpha-1 adrenergic receptors. This selectivity is crucial for understanding receptor function and developing targeted therapies .
Table 1: Affinity of this compound for Alpha-Adrenoceptor Subtypes
Receptor Subtype | Binding Affinity (Ki) |
---|---|
Alpha-1A | High |
Alpha-1B | Moderate |
Alpha-2 | Low |
Hypotensive Effects
Research indicates that this compound has significant hypotensive effects mediated through serotonin-1A (5-HT1A) receptor activation in the central nervous system. Studies have shown that topical application to the ventrolateral medulla results in marked decreases in mean arterial pressure and heart rate .
Case Study: Hypotensive Response in Animal Models
In a study involving chloralose-anesthetized cats, this compound was applied to the ventral surface of the medulla, resulting in a mean arterial pressure reduction of -39 ± 4 mm Hg. The hypotensive effect was blocked by prior administration of a 5-HT1A antagonist, confirming the role of these receptors in mediating the drug's effects .
Applications in Ophthalmology
This compound has been investigated for its potential to reduce intraocular pressure (IOP). In studies with cynomolgus monkeys, it was found to effectively lower IOP and enhance aqueous humor dynamics, suggesting its utility in treating conditions like glaucoma .
Table 2: Effects of this compound on Intraocular Pressure
Study Type | IOP Reduction (mmHg) | Observed Effects |
---|---|---|
Cynomolgus Monkeys | Significant | Increased aqueous humor outflow |
Albino Rabbits | Moderate | Improved ocular perfusion |
Research Applications
The compound is also employed in various research contexts, including:
Mechanism of Action
Urapidil-5-methyl exerts its effects by acting as an alpha-1-adrenoceptor antagonist and a serotonin 5-HT1A receptor agonist . It blocks the vasoconstrictor effect of catecholamines by inhibiting postsynaptic alpha-receptors and modulates the activity of cerebral centers controlling the circulatory system . This dual mechanism of action contributes to its antihypertensive properties and its ability to improve glucose and lipid metabolism .
Comparison with Similar Compounds
Subtype Selectivity Profiles
The pharmacological profiles of 5-methylurapidil and related α1-AR antagonists are summarized below:
Functional Comparisons
- Vascular Smooth Muscle Contraction: In mouse mesenteric arteries, this compound (pA2 = 8.70) and WB4101 (pA2 = 9.40) both inhibit noradrenaline-induced contractions but with differing potencies, reflecting their α1A vs. α1D preferences . In rat aorta, BMY 7378 (α1D-selective) blocks chloroethylclonidine-induced contractions, while this compound shows dual α1A/D activity .
- Renal Vasoconstriction: this compound and BMY 7378 both attenuate noradrenaline-induced renal vasoconstriction in spontaneously hypertensive rats (SHR). However, this compound uniquely enhances phenylephrine responses in renal failure SHR models, suggesting context-dependent interactions .
Prostate Smooth Muscle :
Mechanistic Distinctions
- Biphasic Inhibition: In rat vas deferens, this compound’s inhibition curve is biphasic (IC50 = 0.6 nM and 205 nM), contrasting with monophasic curves for WB4101 and prazosin .
- Tissue-Specific Effects : In renal failure models, this compound enhances phenylephrine-induced vasoconstriction, a response absent with BMY 7378 or chloroethylclonidine .
Research Implications and Limitations
This compound’s dual α1A/D affinity complicates its use in systems expressing both subtypes. For example, in rat aorta, its pKi (8.7) overlaps with α1D-selective compounds like BMY 7378, necessitating complementary approaches (e.g., chloroethylclonidine pretreatment) to isolate α1A responses . Conversely, its α1A selectivity in prostate and cardiac tissues makes it invaluable for studying subtype-specific pathologies like BPH and hypertrophy .
Biological Activity
5-Methylurapidil is a selective antagonist of the α1A-adrenoceptor subtype, primarily studied for its implications in cardiovascular pharmacology. This compound has garnered attention due to its ability to discriminate between various α1-adrenoceptor subtypes, making it a valuable tool in pharmacological research.
This compound exerts its biological activity by selectively binding to α1A-adrenoceptors, inhibiting their activation by endogenous catecholamines like norepinephrine. This selective antagonism has been demonstrated through various studies, including those that utilized radiolabeled ligands to assess binding affinity and functional responses.
Binding Affinity
The binding affinity of this compound for α1-adrenoceptors was assessed using [^3H]prazosin displacement assays across different rat tissues. The results indicated a biphasic displacement pattern, suggesting the presence of both high-affinity and low-affinity binding sites:
Tissue | High-affinity pK_I | Low-affinity pK_I |
---|---|---|
Hippocampus | 9.1 | 7.8 |
Vas deferens | 9.4 | 7.2 |
Heart | 9.3 | 7.5 |
Spleen | Not detected | Low affinity |
Liver | Not detected | Low affinity |
This biphasic response indicates that this compound can differentiate between α1-adrenoceptor subtypes effectively, particularly in tissues where these receptors are expressed variably .
Functional Studies
Functional assays have shown that this compound significantly affects the contractile responses of vascular tissues to norepinephrine. For instance, in studies involving perfused rat kidneys, the presence of 300 nM this compound resulted in biphasic concentration-response curves (CRCs) to norepinephrine, suggesting a complex interaction with receptor subtypes:
- Biphasic CRCs : Indicated the presence of a minor population of receptors resistant to this compound.
- Schild Analysis : The Schild slope was found to be approximately 0.99, indicating competitive antagonism at α1A receptors .
Case Studies and Research Findings
Several studies have highlighted the therapeutic potential of this compound in managing conditions such as hypertension and heart failure:
- Hypertension Management : In animal models, administration of this compound has been shown to reduce blood pressure effectively by inhibiting vasoconstriction mediated by α1A-adrenoceptors.
- Heart Failure : Research indicates that targeting α1A receptors with selective antagonists like this compound may improve cardiac function by reducing afterload and enhancing myocardial perfusion.
Comparative Analysis with Other Antagonists
When comparing the selectivity and efficacy of this compound with other known α1-adrenoceptor antagonists, it stands out due to its high selectivity for the α1A subtype over α1B and α1D subtypes:
Compound | Selectivity for α1A | Comments |
---|---|---|
This compound | High | Best discriminator among subtypes |
Prazosin | Moderate | Non-selective |
WB4101 | Low | Less effective on α1A |
Q & A
Basic Research Questions
Q. What are the primary pharmacological targets of 5-Methylurapidil, and how are they experimentally validated?
- Methodological Answer : this compound is a selective α1-adrenoceptor antagonist. Its affinity for receptor subtypes (α1A, α1B, α1D) is validated using radioligand binding assays (e.g., displacement of [³H]-prazosin) and functional studies in isolated tissues (e.g., rat aorta or portal vein). Competitive inhibition curves and Schild analysis are critical for determining pKi or pKB values .
Q. How should researchers handle storage and preparation of this compound to ensure experimental reproducibility?
- Methodological Answer : Store this compound at room temperature in airtight, light-protected containers. For in vitro studies, prepare stock solutions in DMSO (≤0.1% final concentration to avoid cytotoxicity) and dilute in physiological buffers (e.g., Krebs-Henseleit solution). Verify compound stability via HPLC or mass spectrometry before use .
Q. What experimental models are optimal for studying this compound’s vascular effects?
- Methodological Answer : Isolated rat aortic rings or portal vein preparations are standard models. Pre-contract tissues with phenylephrine (α1-agonist), then apply this compound to assess concentration-dependent relaxation. Control for Ca²⁺ levels, as its presence alters antagonist potency (e.g., pIC50 vs. pKB discrepancies in Ca²⁺-containing vs. Ca²⁺-free media) .
Advanced Research Questions
Q. How do discrepancies in this compound’s pIC50 and pKB values arise, and how should they be resolved?
- Methodological Answer : Discrepancies often stem from experimental conditions (e.g., Ca²⁺ availability). In Ca²⁺-free media, pIC50 aligns with pKB (intrinsic affinity), whereas Ca²⁺ presence introduces functional antagonism. Use Cheng-Prusoff correction to convert pIC50 to pKi under varying conditions. Validate results with multiple antagonists (e.g., WB4101, HV723) to confirm subtype-specific effects .
Q. What strategies differentiate α1-adrenoceptor subtype selectivity of this compound in complex tissues?
- Methodological Answer : Combine knockout models or subtype-selective antagonists (e.g., RS-17053 for α1A) with this compound. Use Scatchard analysis of [³H]-prazosin binding in the presence of this compound to detect allosteric interactions. Functional assays in tissues with known subtype dominance (e.g., rat spleen for α1B) further clarify selectivity .
Q. How can researchers reconcile conflicting data on this compound’s efficacy across in vivo vs. in vitro studies?
- Methodological Answer : In vivo pharmacokinetics (e.g., bioavailability, metabolism) may reduce efficacy compared to in vitro. Perform LC-MS/MS to quantify plasma/tissue concentrations. Use telemetry in conscious animals to monitor hemodynamic responses, ensuring dosing regimens match in vitro EC50 values .
Q. What computational approaches support the design of this compound analogs with improved selectivity?
- Methodological Answer : Employ molecular docking (e.g., AutoDock Vina) to model interactions with α1-subtype binding pockets. Validate with molecular dynamics simulations (e.g., GROMACS) to assess stability. Prioritize analogs with predicted ΔG values < -10 kcal/mol for synthesis and testing .
Q. Data Analysis & Contradiction Management
Q. How should researchers address variability in this compound’s antagonist potency across publications?
- Methodological Answer : Standardize protocols:
- Use consistent tissue sources (e.g., Sprague-Dawley rats).
- Control incubation time (≥30 min for equilibrium).
- Normalize data to internal controls (e.g., maximal phenylephrine response).
- Report pKB values with 95% confidence intervals to enable cross-study comparisons .
Q. What statistical methods are essential for analyzing this compound’s dose-response curves?
- Methodological Answer : Fit data to a logistic equation (e.g., Hill model) using nonlinear regression (GraphPad Prism). Calculate EC50, Hill slope, and maximal response (Emax). For Schild analysis, ensure at least three antagonist concentrations and validate linearity (slope ≈1) .
Q. How can meta-analysis improve understanding of this compound’s pharmacological profile?
- Methodological Answer : Extract data from Scopus or PubMed using keywords (e.g., "this compound AND α1-adrenoceptor"). Apply random-effects models to aggregate pKB values across studies. Assess heterogeneity via I² statistics and publish bias via funnel plots .
Q. Experimental Design & Validation
Q. What controls are critical when testing this compound in vivo for hypertension research?
- Methodological Answer : Include vehicle controls (DMSO/saline), positive controls (e.g., prazosin), and sham-operated animals. Monitor baseline blood pressure and heart rate via radiotelemetry. Use blinded randomization to allocate treatment groups .
Q. How can researchers validate this compound’s off-target effects in complex systems?
Properties
IUPAC Name |
6-[3-[4-(2-methoxyphenyl)piperazin-1-yl]propylamino]-1,3,5-trimethylpyrimidine-2,4-dione | |
---|---|---|
Source | PubChem | |
URL | https://pubchem.ncbi.nlm.nih.gov | |
Description | Data deposited in or computed by PubChem | |
InChI |
InChI=1S/C21H31N5O3/c1-16-19(23(2)21(28)24(3)20(16)27)22-10-7-11-25-12-14-26(15-13-25)17-8-5-6-9-18(17)29-4/h5-6,8-9,22H,7,10-15H2,1-4H3 | |
Source | PubChem | |
URL | https://pubchem.ncbi.nlm.nih.gov | |
Description | Data deposited in or computed by PubChem | |
InChI Key |
HIHZDNKKIUQQSC-UHFFFAOYSA-N | |
Source | PubChem | |
URL | https://pubchem.ncbi.nlm.nih.gov | |
Description | Data deposited in or computed by PubChem | |
Canonical SMILES |
CC1=C(N(C(=O)N(C1=O)C)C)NCCCN2CCN(CC2)C3=CC=CC=C3OC | |
Source | PubChem | |
URL | https://pubchem.ncbi.nlm.nih.gov | |
Description | Data deposited in or computed by PubChem | |
Molecular Formula |
C21H31N5O3 | |
Source | PubChem | |
URL | https://pubchem.ncbi.nlm.nih.gov | |
Description | Data deposited in or computed by PubChem | |
DSSTOX Substance ID |
DTXSID60956150 | |
Record name | 6-((3-(4-(2-Methoxyphenyl)-1-piperazinyl)propyl)amino)-1,3,5-trimethyl-2,4(1H,3H)-pyrimidinedione | |
Source | EPA DSSTox | |
URL | https://comptox.epa.gov/dashboard/DTXSID60956150 | |
Description | DSSTox provides a high quality public chemistry resource for supporting improved predictive toxicology. | |
Molecular Weight |
401.5 g/mol | |
Source | PubChem | |
URL | https://pubchem.ncbi.nlm.nih.gov | |
Description | Data deposited in or computed by PubChem | |
CAS No. |
34661-85-3 | |
Record name | 5-Methylurapidil | |
Source | CAS Common Chemistry | |
URL | https://commonchemistry.cas.org/detail?cas_rn=34661-85-3 | |
Description | CAS Common Chemistry is an open community resource for accessing chemical information. Nearly 500,000 chemical substances from CAS REGISTRY cover areas of community interest, including common and frequently regulated chemicals, and those relevant to high school and undergraduate chemistry classes. This chemical information, curated by our expert scientists, is provided in alignment with our mission as a division of the American Chemical Society. | |
Explanation | The data from CAS Common Chemistry is provided under a CC-BY-NC 4.0 license, unless otherwise stated. | |
Record name | 5-Methylurapidil | |
Source | ChemIDplus | |
URL | https://pubchem.ncbi.nlm.nih.gov/substance/?source=chemidplus&sourceid=0034661853 | |
Description | ChemIDplus is a free, web search system that provides access to the structure and nomenclature authority files used for the identification of chemical substances cited in National Library of Medicine (NLM) databases, including the TOXNET system. | |
Record name | 6-((3-(4-(2-Methoxyphenyl)-1-piperazinyl)propyl)amino)-1,3,5-trimethyl-2,4(1H,3H)-pyrimidinedione | |
Source | EPA DSSTox | |
URL | https://comptox.epa.gov/dashboard/DTXSID60956150 | |
Description | DSSTox provides a high quality public chemistry resource for supporting improved predictive toxicology. | |
Record name | 5-METHYLURAPIDIL | |
Source | FDA Global Substance Registration System (GSRS) | |
URL | https://gsrs.ncats.nih.gov/ginas/app/beta/substances/1HLS600135 | |
Description | The FDA Global Substance Registration System (GSRS) enables the efficient and accurate exchange of information on what substances are in regulated products. Instead of relying on names, which vary across regulatory domains, countries, and regions, the GSRS knowledge base makes it possible for substances to be defined by standardized, scientific descriptions. | |
Explanation | Unless otherwise noted, the contents of the FDA website (www.fda.gov), both text and graphics, are not copyrighted. They are in the public domain and may be republished, reprinted and otherwise used freely by anyone without the need to obtain permission from FDA. Credit to the U.S. Food and Drug Administration as the source is appreciated but not required. | |
Retrosynthesis Analysis
AI-Powered Synthesis Planning: Our tool employs the Template_relevance Pistachio, Template_relevance Bkms_metabolic, Template_relevance Pistachio_ringbreaker, Template_relevance Reaxys, Template_relevance Reaxys_biocatalysis model, leveraging a vast database of chemical reactions to predict feasible synthetic routes.
One-Step Synthesis Focus: Specifically designed for one-step synthesis, it provides concise and direct routes for your target compounds, streamlining the synthesis process.
Accurate Predictions: Utilizing the extensive PISTACHIO, BKMS_METABOLIC, PISTACHIO_RINGBREAKER, REAXYS, REAXYS_BIOCATALYSIS database, our tool offers high-accuracy predictions, reflecting the latest in chemical research and data.
Strategy Settings
Precursor scoring | Relevance Heuristic |
---|---|
Min. plausibility | 0.01 |
Model | Template_relevance |
Template Set | Pistachio/Bkms_metabolic/Pistachio_ringbreaker/Reaxys/Reaxys_biocatalysis |
Top-N result to add to graph | 6 |
Feasible Synthetic Routes
Disclaimer and Information on In-Vitro Research Products
Please be aware that all articles and product information presented on BenchChem are intended solely for informational purposes. The products available for purchase on BenchChem are specifically designed for in-vitro studies, which are conducted outside of living organisms. In-vitro studies, derived from the Latin term "in glass," involve experiments performed in controlled laboratory settings using cells or tissues. It is important to note that these products are not categorized as medicines or drugs, and they have not received approval from the FDA for the prevention, treatment, or cure of any medical condition, ailment, or disease. We must emphasize that any form of bodily introduction of these products into humans or animals is strictly prohibited by law. It is essential to adhere to these guidelines to ensure compliance with legal and ethical standards in research and experimentation.