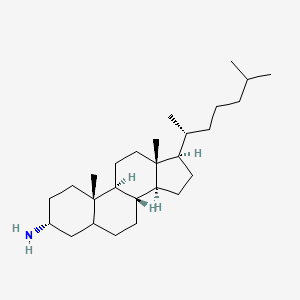
(3alpha,5alpha)-Cholestan-3-amine
Overview
Description
3α-Aminocholestane is a synthetic organic compound known for its role as an inhibitor of inositol polyphosphate-5-phosphatase D, commonly referred to as SH2 domain-containing inositol-5’-phosphatase 1 (SHIP1). This enzyme plays a crucial role in controlling immune regulatory cell numbers in vivo .
Mechanism of Action
Target of Action
The primary target of 3|A-Aminocholestane is the SH2 domain-containing inositol-5’-phosphatase 1 (SHIP1) . SHIP1 is an enzyme that plays a crucial role in controlling immune regulatory cell numbers in vivo .
Mode of Action
3|A-Aminocholestane acts as a selective inhibitor of SHIP1 . It interacts with SHIP1 and inhibits its activity, with an IC50 value of approximately 2.5 μM . This inhibition leads to changes in the activity of immune regulatory cells.
Biochemical Pathways
The inhibition of SHIP1 by 3|A-Aminocholestane affects the phosphatidylinositol signaling pathway . SHIP1 normally dephosphorylates the 5-position of PI (3,4,5)P3, generating PI (3,4)P2 . By inhibiting SHIP1, 3|A-Aminocholestane disrupts this process, leading to alterations in the downstream effects of the phosphatidylinositol signaling pathway.
Result of Action
The inhibition of SHIP1 by 3|A-Aminocholestane has several molecular and cellular effects. It has been shown to reduce cell viability in OPM2 multiple myeloma (MM) cells in a concentration-dependent manner . It also halts the cell cycle at the G0/G1 or G2/M stages in different cell lines . Furthermore, it induces apoptosis via activation of caspase-3, caspase-9, and poly (ADP-ribose) polymerase (PARP) in OPM2 cells .
Biochemical Analysis
Biochemical Properties
(3alpha,5alpha)-Cholestan-3-amine plays a significant role in biochemical reactions, particularly in the modulation of membrane fluidity and signaling pathways. This compound interacts with various enzymes, proteins, and other biomolecules. For instance, it has been shown to interact with GABA(A) receptors, which are crucial for inhibitory neurotransmission in the brain . The nature of these interactions involves binding to specific receptor subunits, influencing their activity and, consequently, the overall inhibitory tone in neuronal circuits.
Cellular Effects
The effects of this compound on various cell types and cellular processes are profound. In neuronal cells, this compound influences cell signaling pathways, gene expression, and cellular metabolism. It modulates the activity of GABA(A) receptors, leading to changes in synaptic transmission and neuronal excitability . Additionally, this compound has been implicated in the regulation of lipid metabolism and membrane dynamics in other cell types, affecting processes such as cell proliferation and apoptosis.
Molecular Mechanism
At the molecular level, this compound exerts its effects through binding interactions with biomolecules, enzyme inhibition or activation, and changes in gene expression. The compound binds to specific sites on GABA(A) receptors, altering their conformation and activity . This binding can either enhance or inhibit receptor function, depending on the context and the specific receptor subunits involved. Additionally, this compound can influence the expression of genes related to lipid metabolism and cell signaling pathways, further modulating cellular functions.
Temporal Effects in Laboratory Settings
In laboratory settings, the effects of this compound can change over time. The stability and degradation of this compound are critical factors that influence its long-term effects on cellular function. Studies have shown that this compound remains stable under physiological conditions, allowing for sustained interactions with target biomolecules . Prolonged exposure to this compound can lead to adaptive changes in cellular processes, such as receptor desensitization and alterations in gene expression patterns.
Dosage Effects in Animal Models
The effects of this compound vary with different dosages in animal models. At low doses, this compound can enhance inhibitory neurotransmission and improve cognitive functions by modulating GABA(A) receptor activity . At high doses, this compound may exhibit toxic or adverse effects, such as neurotoxicity and disruption of lipid homeostasis. Threshold effects have been observed, where specific dosages elicit distinct cellular responses, highlighting the importance of dose optimization in therapeutic applications.
Metabolic Pathways
This compound is involved in several metabolic pathways, including those related to lipid metabolism and neurotransmitter synthesis. It interacts with enzymes such as cholesterol oxidase and acetyl-CoA acetyltransferase, influencing the flux of metabolites through these pathways . These interactions can affect the levels of key metabolites, such as cholesterol and acetyl-CoA, thereby modulating cellular energy balance and signaling processes.
Transport and Distribution
The transport and distribution of this compound within cells and tissues are mediated by specific transporters and binding proteins. This compound can be transported across cell membranes via lipid transporters and can bind to intracellular proteins that facilitate its localization to specific cellular compartments . The distribution of this compound within tissues can influence its overall bioavailability and efficacy in modulating cellular functions.
Subcellular Localization
This compound exhibits distinct subcellular localization patterns, which can affect its activity and function. This compound is often localized to lipid rafts and membrane microdomains, where it interacts with membrane-bound receptors and signaling proteins . Post-translational modifications, such as phosphorylation and acetylation, can also influence the targeting and localization of this compound to specific organelles, such as the endoplasmic reticulum and mitochondria.
Preparation Methods
Synthetic Routes and Reaction Conditions
3α-Aminocholestane can be synthesized through a multi-step process starting from cholesterol. The key steps involve:
Oxidation: Cholesterol is oxidized to form cholest-4-en-3-one.
Reduction: The ketone group is reduced to form cholestan-3β-ol.
Amination: The hydroxyl group is then converted to an amine group through a series of reactions involving tosylation and nucleophilic substitution.
Industrial Production Methods
While specific industrial production methods for 3α-Aminocholestane are not extensively documented, the general approach involves large-scale synthesis using the aforementioned synthetic routes. The process is optimized for yield and purity, often involving purification steps such as recrystallization and chromatography.
Chemical Reactions Analysis
Types of Reactions
3α-Aminocholestane undergoes several types of chemical reactions, including:
Oxidation: The amine group can be oxidized to form corresponding oximes or nitro compounds.
Reduction: The compound can be reduced to form secondary or tertiary amines.
Substitution: The amine group can participate in nucleophilic substitution reactions to form various derivatives.
Common Reagents and Conditions
Oxidation: Common oxidizing agents include potassium permanganate and chromium trioxide.
Reduction: Reducing agents such as lithium aluminum hydride and sodium borohydride are used.
Substitution: Reagents like tosyl chloride and sodium azide are employed for nucleophilic substitution reactions.
Major Products
The major products formed from these reactions include oximes, nitro compounds, secondary amines, tertiary amines, and various substituted derivatives.
Scientific Research Applications
3α-Aminocholestane has a wide range of scientific research applications:
Chemistry: Used as a reagent in organic synthesis and as a precursor for other complex molecules.
Biology: Studied for its role in modulating immune responses by inhibiting SHIP1.
Industry: Utilized in the development of new pharmaceuticals and as a tool in biochemical research.
Comparison with Similar Compounds
Similar Compounds
3β-Aminocholestane: Similar structure but differs in the position of the amine group.
Cholestane: Lacks the amine group, serving as a simpler analog.
Cholestan-3-one: Contains a ketone group instead of an amine.
Uniqueness
3α-Aminocholestane is unique due to its selective inhibition of SHIP1, which distinguishes it from other similar compounds that may not exhibit the same level of specificity or biological activity .
Properties
IUPAC Name |
(3R,5S,8R,9S,10S,13R,14S,17R)-10,13-dimethyl-17-[(2R)-6-methylheptan-2-yl]-2,3,4,5,6,7,8,9,11,12,14,15,16,17-tetradecahydro-1H-cyclopenta[a]phenanthren-3-amine | |
---|---|---|
Source | PubChem | |
URL | https://pubchem.ncbi.nlm.nih.gov | |
Description | Data deposited in or computed by PubChem | |
InChI |
InChI=1S/C27H49N/c1-18(2)7-6-8-19(3)23-11-12-24-22-10-9-20-17-21(28)13-15-26(20,4)25(22)14-16-27(23,24)5/h18-25H,6-17,28H2,1-5H3/t19-,20+,21-,22+,23-,24+,25+,26+,27-/m1/s1 | |
Source | PubChem | |
URL | https://pubchem.ncbi.nlm.nih.gov | |
Description | Data deposited in or computed by PubChem | |
InChI Key |
RJNGJYWAIUJHOJ-FBVYSKEZSA-N | |
Source | PubChem | |
URL | https://pubchem.ncbi.nlm.nih.gov | |
Description | Data deposited in or computed by PubChem | |
Canonical SMILES |
CC(C)CCCC(C)C1CCC2C1(CCC3C2CCC4C3(CCC(C4)N)C)C | |
Source | PubChem | |
URL | https://pubchem.ncbi.nlm.nih.gov | |
Description | Data deposited in or computed by PubChem | |
Isomeric SMILES |
C[C@H](CCCC(C)C)[C@H]1CC[C@@H]2[C@@]1(CC[C@H]3[C@H]2CC[C@@H]4[C@@]3(CC[C@H](C4)N)C)C | |
Source | PubChem | |
URL | https://pubchem.ncbi.nlm.nih.gov | |
Description | Data deposited in or computed by PubChem | |
Molecular Formula |
C27H49N | |
Source | PubChem | |
URL | https://pubchem.ncbi.nlm.nih.gov | |
Description | Data deposited in or computed by PubChem | |
Molecular Weight |
387.7 g/mol | |
Source | PubChem | |
URL | https://pubchem.ncbi.nlm.nih.gov | |
Description | Data deposited in or computed by PubChem | |
Retrosynthesis Analysis
AI-Powered Synthesis Planning: Our tool employs the Template_relevance Pistachio, Template_relevance Bkms_metabolic, Template_relevance Pistachio_ringbreaker, Template_relevance Reaxys, Template_relevance Reaxys_biocatalysis model, leveraging a vast database of chemical reactions to predict feasible synthetic routes.
One-Step Synthesis Focus: Specifically designed for one-step synthesis, it provides concise and direct routes for your target compounds, streamlining the synthesis process.
Accurate Predictions: Utilizing the extensive PISTACHIO, BKMS_METABOLIC, PISTACHIO_RINGBREAKER, REAXYS, REAXYS_BIOCATALYSIS database, our tool offers high-accuracy predictions, reflecting the latest in chemical research and data.
Strategy Settings
Precursor scoring | Relevance Heuristic |
---|---|
Min. plausibility | 0.01 |
Model | Template_relevance |
Template Set | Pistachio/Bkms_metabolic/Pistachio_ringbreaker/Reaxys/Reaxys_biocatalysis |
Top-N result to add to graph | 6 |
Feasible Synthetic Routes
Disclaimer and Information on In-Vitro Research Products
Please be aware that all articles and product information presented on BenchChem are intended solely for informational purposes. The products available for purchase on BenchChem are specifically designed for in-vitro studies, which are conducted outside of living organisms. In-vitro studies, derived from the Latin term "in glass," involve experiments performed in controlled laboratory settings using cells or tissues. It is important to note that these products are not categorized as medicines or drugs, and they have not received approval from the FDA for the prevention, treatment, or cure of any medical condition, ailment, or disease. We must emphasize that any form of bodily introduction of these products into humans or animals is strictly prohibited by law. It is essential to adhere to these guidelines to ensure compliance with legal and ethical standards in research and experimentation.