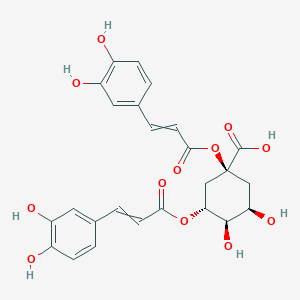
1,3-Dicaffeoylquinic acid
Overview
Description
1,3-Dicaffeoylquinic acid is a naturally occurring polyphenolic compound found in various medicinal plants such as Echinacea species and Hedera helix . It is an ester composed of quinic acid and two caffeoyl acid moieties. This compound is one of the six known dicaffeoylquinic acid isomers, which include 1,3-, 1,4-, 1,5-, 3,4-, 3,5-, and 4,5-dicaffeoylquinic acid . These compounds are known for their antioxidative, anti-inflammatory, antimicrobial, hypoglycaemic, cardiovascular protective, neuroprotective, and hepatoprotective effects .
Mechanism of Action
1,3-O-Dicaffeoylquinic acid, also known as 1,3-Dicaffeoylquinic acid or 1,5-Dicaffeoylquinic acid, is a caffeoylquinic acid derivative that exhibits antioxidant activity and radical scavenging activity .
Target of Action
The primary targets of 1,3-O-Dicaffeoylquinic acid are phosphoinositide 3-kinase (PI3K)/Akt and extracellular regulated protein kinase 1/2 (Erk1/2) pathways . These pathways play a crucial role in cell survival, growth, and proliferation.
Mode of Action
1,3-O-Dicaffeoylquinic acid shows increased neuronal cell viability against Aβ(42) toxicity in a concentration-dependent manner in neurons . It activates both PI3K/Akt and Erk1/2 pathways, stimulating their upstream tyrosine kinase A (Trk A) . Its anti-apoptotic potential is related to the enhanced inactivating phosphorylation of glycogen synthase kinase 3β (GSK3β) and the modulation of expression of apoptosis-related protein Bcl-2/Bax .
Biochemical Pathways
The compound’s action on the PI3K/Akt and Erk1/2 pathways leads to a series of downstream effects. For instance, it significantly increases cell viability before oxygen-glucose deprivation/reperfusion (OGD/reperfusion), and prevents the depletion of glutathione (GSH) under OGD/reperfusion insult . It also induces nuclear translocation of Nrf2 in OGD/reperfusion treated astrocytes, and induces increased glutamate-cysteine ligase (GCL) activity .
Pharmacokinetics
In terms of ADME properties, 1,3-O-Dicaffeoylquinic acid and its metabolite 1-O-ABL are absorbed very quickly in Wistar rats . The maximum plasma concentrations for this compound and 1-O-ABL are 44.5 ± 7.1 and 19.1 ± 6.9 ng/mL, respectively .
Result of Action
The molecular and cellular effects of 1,3-O-Dicaffeoylquinic acid’s action include increased neuronal cell viability, enhanced inactivating phosphorylation of GSK3β, modulation of Bcl-2/Bax expression, prevention of GSH depletion, and increased GCL activity .
Biochemical Analysis
Biochemical Properties
1,3-O-Dicaffeoylquinic acid interacts with various enzymes, proteins, and other biomolecules. It has been shown to inhibit the activity of drug transporter proteins in vitro . It also inhibits the activity of matrix metalloproteinases and proinflammatory cytokines such as IL-1β . The compound’s role in biochemical reactions is influenced by its structure, which includes two caffeic acid molecules linked via ester bonds to one quinic acid molecule .
Cellular Effects
1,3-O-Dicaffeoylquinic acid has various effects on different types of cells and cellular processes. It has been shown to have low toxicity and exhibits therapeutic potential by regulating mitochondrial membrane potential, reducing cell apoptosis, and mitigating Aβ (1–40)-induced cellular damage . It also influences cell function by impacting cell signaling pathways, gene expression, and cellular metabolism .
Molecular Mechanism
The molecular mechanism of 1,3-O-Dicaffeoylquinic acid involves its interactions with biomolecules at the molecular level. It disrupts Aβ (1–40) self-aggregation by interacting with specific phenolic hydroxyl and amino acid residues, particularly at Met-35 in Aβ (1–40) . It exerts its effects through binding interactions with biomolecules, enzyme inhibition or activation, and changes in gene expression .
Metabolic Pathways
1,3-O-Dicaffeoylquinic acid is involved in various metabolic pathways. It is a part of the phenylpropanoid biosynthesis pathway
Preparation Methods
Synthetic Routes and Reaction Conditions
1,3-Dicaffeoylquinic acid can be synthesized through the esterification of quinic acid with caffeic acid. The reaction typically involves the use of a coupling agent such as dicyclohexylcarbodiimide (DCC) and a catalyst like 4-dimethylaminopyridine (DMAP) in an organic solvent such as dichloromethane . The reaction is carried out at room temperature and monitored by thin-layer chromatography (TLC) until completion. The product is then purified by column chromatography to obtain pure this compound.
Industrial Production Methods
Industrial production of this compound often involves extraction from natural sources. Plants rich in dicaffeoylquinic acids, such as Echinacea and coffee, are processed to extract the compound. The extraction process typically involves solvent extraction using solvents like methanol or ethanol, followed by purification steps such as liquid-liquid extraction and chromatography .
Chemical Reactions Analysis
Types of Reactions
1,3-Dicaffeoylquinic acid undergoes various chemical reactions, including:
Oxidation: The compound can be oxidized to form quinones and other oxidation products.
Reduction: Reduction of this compound can lead to the formation of dihydro derivatives.
Substitution: The hydroxyl groups in this compound can undergo substitution reactions with various reagents.
Common Reagents and Conditions
Reduction: Sodium borohydride; reactions are usually performed in methanol or ethanol at room temperature.
Substitution: Acetic anhydride, pyridine; reactions are carried out in organic solvents like dichloromethane at room temperature.
Major Products Formed
Oxidation: Quinones and other oxidation products.
Reduction: Dihydro derivatives.
Substitution: Acetylated derivatives.
Scientific Research Applications
1,3-Dicaffeoylquinic acid has a wide range of scientific research applications:
Comparison with Similar Compounds
1,3-Dicaffeoylquinic acid is one of several dicaffeoylquinic acid isomers. Similar compounds include:
- 1,4-Dicaffeoylquinic acid
- 1,5-Dicaffeoylquinic acid
- 3,4-Dicaffeoylquinic acid
- 3,5-Dicaffeoylquinic acid
- 4,5-Dicaffeoylquinic acid
- Isochlorogenic acid A, B, and C
- Caftaric acid
- Chicoric acid
These compounds share similar structures but differ in the positions of the caffeoyl groups on the quinic acid ring. The unique positioning of the caffeoyl groups in this compound contributes to its specific biological activities and pharmacological properties .
Properties
IUPAC Name |
(1R,3R,4S,5R)-1,3-bis[[(E)-3-(3,4-dihydroxyphenyl)prop-2-enoyl]oxy]-4,5-dihydroxycyclohexane-1-carboxylic acid | |
---|---|---|
Source | PubChem | |
URL | https://pubchem.ncbi.nlm.nih.gov | |
Description | Data deposited in or computed by PubChem | |
InChI |
InChI=1S/C25H24O12/c26-15-5-1-13(9-17(15)28)3-7-21(31)36-20-12-25(24(34)35,11-19(30)23(20)33)37-22(32)8-4-14-2-6-16(27)18(29)10-14/h1-10,19-20,23,26-30,33H,11-12H2,(H,34,35)/b7-3+,8-4+/t19-,20-,23+,25-/m1/s1 | |
Source | PubChem | |
URL | https://pubchem.ncbi.nlm.nih.gov | |
Description | Data deposited in or computed by PubChem | |
InChI Key |
YDDUMTOHNYZQPO-RVXRWRFUSA-N | |
Source | PubChem | |
URL | https://pubchem.ncbi.nlm.nih.gov | |
Description | Data deposited in or computed by PubChem | |
Canonical SMILES |
C1C(C(C(CC1(C(=O)O)OC(=O)C=CC2=CC(=C(C=C2)O)O)OC(=O)C=CC3=CC(=C(C=C3)O)O)O)O | |
Source | PubChem | |
URL | https://pubchem.ncbi.nlm.nih.gov | |
Description | Data deposited in or computed by PubChem | |
Isomeric SMILES |
C1[C@H]([C@@H]([C@@H](C[C@]1(C(=O)O)OC(=O)/C=C/C2=CC(=C(C=C2)O)O)OC(=O)/C=C/C3=CC(=C(C=C3)O)O)O)O | |
Source | PubChem | |
URL | https://pubchem.ncbi.nlm.nih.gov | |
Description | Data deposited in or computed by PubChem | |
Molecular Formula |
C25H24O12 | |
Source | PubChem | |
URL | https://pubchem.ncbi.nlm.nih.gov | |
Description | Data deposited in or computed by PubChem | |
DSSTOX Substance ID |
DTXSID301309674 | |
Record name | 1,5-Di-O-caffeoylquinic acid | |
Source | EPA DSSTox | |
URL | https://comptox.epa.gov/dashboard/DTXSID301309674 | |
Description | DSSTox provides a high quality public chemistry resource for supporting improved predictive toxicology. | |
Molecular Weight |
516.4 g/mol | |
Source | PubChem | |
URL | https://pubchem.ncbi.nlm.nih.gov | |
Description | Data deposited in or computed by PubChem | |
CAS No. |
212891-05-9, 30964-13-7 | |
Record name | 1,5-Di-O-caffeoylquinic acid | |
Source | CAS Common Chemistry | |
URL | https://commonchemistry.cas.org/detail?cas_rn=212891-05-9 | |
Description | CAS Common Chemistry is an open community resource for accessing chemical information. Nearly 500,000 chemical substances from CAS REGISTRY cover areas of community interest, including common and frequently regulated chemicals, and those relevant to high school and undergraduate chemistry classes. This chemical information, curated by our expert scientists, is provided in alignment with our mission as a division of the American Chemical Society. | |
Explanation | The data from CAS Common Chemistry is provided under a CC-BY-NC 4.0 license, unless otherwise stated. | |
Record name | Cynarine [INN] | |
Source | ChemIDplus | |
URL | https://pubchem.ncbi.nlm.nih.gov/substance/?source=chemidplus&sourceid=0030964137 | |
Description | ChemIDplus is a free, web search system that provides access to the structure and nomenclature authority files used for the identification of chemical substances cited in National Library of Medicine (NLM) databases, including the TOXNET system. | |
Record name | Cynarine [INN:WHO-DD] | |
Source | ChemIDplus | |
URL | https://pubchem.ncbi.nlm.nih.gov/substance/?source=chemidplus&sourceid=0212891059 | |
Description | ChemIDplus is a free, web search system that provides access to the structure and nomenclature authority files used for the identification of chemical substances cited in National Library of Medicine (NLM) databases, including the TOXNET system. | |
Record name | 1,5-Di-O-caffeoylquinic acid | |
Source | EPA DSSTox | |
URL | https://comptox.epa.gov/dashboard/DTXSID301309674 | |
Description | DSSTox provides a high quality public chemistry resource for supporting improved predictive toxicology. | |
Record name | CYNARINE | |
Source | FDA Global Substance Registration System (GSRS) | |
URL | https://gsrs.ncats.nih.gov/ginas/app/beta/substances/85D81U9JAV | |
Description | The FDA Global Substance Registration System (GSRS) enables the efficient and accurate exchange of information on what substances are in regulated products. Instead of relying on names, which vary across regulatory domains, countries, and regions, the GSRS knowledge base makes it possible for substances to be defined by standardized, scientific descriptions. | |
Explanation | Unless otherwise noted, the contents of the FDA website (www.fda.gov), both text and graphics, are not copyrighted. They are in the public domain and may be republished, reprinted and otherwise used freely by anyone without the need to obtain permission from FDA. Credit to the U.S. Food and Drug Administration as the source is appreciated but not required. | |
Record name | 1,5-Dicaffeoylquinic acid | |
Source | Human Metabolome Database (HMDB) | |
URL | http://www.hmdb.ca/metabolites/HMDB0030093 | |
Description | The Human Metabolome Database (HMDB) is a freely available electronic database containing detailed information about small molecule metabolites found in the human body. | |
Explanation | HMDB is offered to the public as a freely available resource. Use and re-distribution of the data, in whole or in part, for commercial purposes requires explicit permission of the authors and explicit acknowledgment of the source material (HMDB) and the original publication (see the HMDB citing page). We ask that users who download significant portions of the database cite the HMDB paper in any resulting publications. | |
Retrosynthesis Analysis
AI-Powered Synthesis Planning: Our tool employs the Template_relevance Pistachio, Template_relevance Bkms_metabolic, Template_relevance Pistachio_ringbreaker, Template_relevance Reaxys, Template_relevance Reaxys_biocatalysis model, leveraging a vast database of chemical reactions to predict feasible synthetic routes.
One-Step Synthesis Focus: Specifically designed for one-step synthesis, it provides concise and direct routes for your target compounds, streamlining the synthesis process.
Accurate Predictions: Utilizing the extensive PISTACHIO, BKMS_METABOLIC, PISTACHIO_RINGBREAKER, REAXYS, REAXYS_BIOCATALYSIS database, our tool offers high-accuracy predictions, reflecting the latest in chemical research and data.
Strategy Settings
Precursor scoring | Relevance Heuristic |
---|---|
Min. plausibility | 0.01 |
Model | Template_relevance |
Template Set | Pistachio/Bkms_metabolic/Pistachio_ringbreaker/Reaxys/Reaxys_biocatalysis |
Top-N result to add to graph | 6 |
Feasible Synthetic Routes
Disclaimer and Information on In-Vitro Research Products
Please be aware that all articles and product information presented on BenchChem are intended solely for informational purposes. The products available for purchase on BenchChem are specifically designed for in-vitro studies, which are conducted outside of living organisms. In-vitro studies, derived from the Latin term "in glass," involve experiments performed in controlled laboratory settings using cells or tissues. It is important to note that these products are not categorized as medicines or drugs, and they have not received approval from the FDA for the prevention, treatment, or cure of any medical condition, ailment, or disease. We must emphasize that any form of bodily introduction of these products into humans or animals is strictly prohibited by law. It is essential to adhere to these guidelines to ensure compliance with legal and ethical standards in research and experimentation.