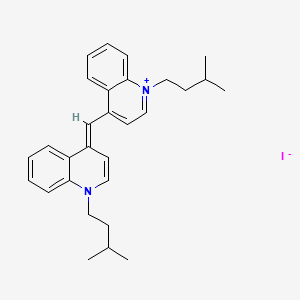
Cyanine
Overview
Description
Cyanines are a synthetic dye family belonging to the polymethine group . They are a conjugated system between two nitrogen atoms . Cyanines cover the electromagnetic spectrum from near IR to UV . They are commonly used in biological labeling .
Synthesis Analysis
Cyanine dyes are synthesized from 2, 3, 5, or 7-methine structures with reactive groups on either one or both of the nitrogen ends so that they can be chemically linked to either nucleic acids or protein molecules . A straightforward synthetic route for the design and synthesis of diverse heterobifunctional cyanine 5 dyes has been presented .
Molecular Structure Analysis
Cyanines have been classified into Streptocyanines or open chain cyanines, Hemicyanines, and Closed chain cyanines . Both nitrogens may each be independently part of a heteroaromatic moiety, such as pyrrole, imidazole, thiazole, pyridine, quinoline, indole, benzothiazole, etc .
Chemical Reactions Analysis
Cyanine dyes are known to form large-scale aggregates of various morphologies via spontaneous self-assembly in aqueous solution . A phototruncation reaction converts heptamethine cyanine into pentamethine cyanine, and then into trimethine cyanine, shifting the light absorbance spectra .
Physical And Chemical Properties Analysis
Cyanine dyes exhibit a strong shift of the monomer (M) absorption band, upon aggregation in aqueous solution, towards a longer wavelength . Dyes which exhibit this bathochromic (red) shift, resulting in a sharp narrow absorption band, are termed J-aggregates .
Mechanism of Action
Target of Action
Cyanine dyes are primarily used as bioprobes in the fields of medicine, genetics, and environment . They are often used for fluorescent labeling of biological macromolecules, disease diagnosis, immunoassay, and DNA detection . Cyanine dyes, specifically Cy3 and Cy5, have been used as vectors for targeting and delivering cargoes to mitochondria in cancer cells . This selective binding to albumin results in enhanced optical properties and improved biosafety .
Mode of Action
Cyanine dyes are a conjugated system between two nitrogen atoms . In each resonance structure, exactly one nitrogen atom is oxidized to an iminium . They form part of a nitrogenous heterocyclic system . Cyanine dyes can increase cytotoxicity by up to 1000-fold when conjugated to the dyes .
Biochemical Pathways
Cyanine dyes are widely used as fluorescent probes in biophysics and medical biochemistry due to their unique photophysical and photochemical properties . They are used in various studies such as RNA localization, molecular interaction studies by fluorescence resonance energy transfer (FRET), and fluorescent immunoassays . The application of cyanine dyes as bioprobes with different identification elements, including enzymes, organelles, immunity, and DNAs, was mainly summarized .
Pharmacokinetics
The current issues with cyanine dyes include aggregation-induced quenching, poor photostability, and short in vivo circulation time . Encapsulating cyanine dyes with albumin, whether exogenous or endogenous, has been proven to be an effective strategy for improving their brightness and pharmacokinetics . In detail, the chloride-containing (Cl-containing) cyanine dyes have been found to selectively bind to albumin to achieve site-specific albumin tagging, resulting in enhanced optical properties and improved biosafety .
Result of Action
Cyanine dyes are known for their ability to increase the sensitivity range of photographic emulsions, i.e., to increase the range of wavelengths which will form an image on the film, making the film panchromatic . They are also used in CD-R and DVD-R media . The ones used are mostly green or light blue color, and are chemically unstable . Recent cyanine discs contain stabilizers, typically a metal atom bonded to the cyanine molecule, that slow the deterioration significantly .
Action Environment
Cyanine dyes are sensitive to their electronic environment . Changes in the conformation of the protein they are attached to will produce either enhancement or quenching of the emission . The rate of this change can be measured to determine enzyme kinetic parameters . The process known as “photoblueing” can confound experimental results . The new findings could help manipulate reaction conditions to avoid the process or to harness it .
Future Directions
properties
IUPAC Name |
(4E)-1-(3-methylbutyl)-4-[[1-(3-methylbutyl)quinolin-1-ium-4-yl]methylidene]quinoline;iodide | |
---|---|---|
Source | PubChem | |
URL | https://pubchem.ncbi.nlm.nih.gov | |
Description | Data deposited in or computed by PubChem | |
InChI |
InChI=1S/C29H35N2.HI/c1-22(2)13-17-30-19-15-24(26-9-5-7-11-28(26)30)21-25-16-20-31(18-14-23(3)4)29-12-8-6-10-27(25)29;/h5-12,15-16,19-23H,13-14,17-18H2,1-4H3;1H/q+1;/p-1 | |
Source | PubChem | |
URL | https://pubchem.ncbi.nlm.nih.gov | |
Description | Data deposited in or computed by PubChem | |
InChI Key |
QGKMIGUHVLGJBR-UHFFFAOYSA-M | |
Source | PubChem | |
URL | https://pubchem.ncbi.nlm.nih.gov | |
Description | Data deposited in or computed by PubChem | |
Canonical SMILES |
CC(C)CCN1C=CC(=CC2=CC=[N+](C3=CC=CC=C23)CCC(C)C)C4=CC=CC=C41.[I-] | |
Source | PubChem | |
URL | https://pubchem.ncbi.nlm.nih.gov | |
Description | Data deposited in or computed by PubChem | |
Isomeric SMILES |
CC(C)CCN1C=C/C(=C\C2=CC=[N+](C3=CC=CC=C23)CCC(C)C)/C4=CC=CC=C41.[I-] | |
Source | PubChem | |
URL | https://pubchem.ncbi.nlm.nih.gov | |
Description | Data deposited in or computed by PubChem | |
Molecular Formula |
C29H35IN2 | |
Source | PubChem | |
URL | https://pubchem.ncbi.nlm.nih.gov | |
Description | Data deposited in or computed by PubChem | |
Molecular Weight |
538.5 g/mol | |
Source | PubChem | |
URL | https://pubchem.ncbi.nlm.nih.gov | |
Description | Data deposited in or computed by PubChem | |
Product Name |
Cyanine | |
CAS RN |
523-42-2 | |
Record name | Quinoline blue | |
Source | CAS Common Chemistry | |
URL | https://commonchemistry.cas.org/detail?cas_rn=523-42-2 | |
Description | CAS Common Chemistry is an open community resource for accessing chemical information. Nearly 500,000 chemical substances from CAS REGISTRY cover areas of community interest, including common and frequently regulated chemicals, and those relevant to high school and undergraduate chemistry classes. This chemical information, curated by our expert scientists, is provided in alignment with our mission as a division of the American Chemical Society. | |
Explanation | The data from CAS Common Chemistry is provided under a CC-BY-NC 4.0 license, unless otherwise stated. | |
Record name | AI 3-19500 | |
Source | ChemIDplus | |
URL | https://pubchem.ncbi.nlm.nih.gov/substance/?source=chemidplus&sourceid=0000523422 | |
Description | ChemIDplus is a free, web search system that provides access to the structure and nomenclature authority files used for the identification of chemical substances cited in National Library of Medicine (NLM) databases, including the TOXNET system. | |
Record name | 1-(3-methylbutyl)-4-[[1-(3-methylbutyl)-4(1H)-quinolylidene]methyl]quinolinium iodide | |
Source | European Chemicals Agency (ECHA) | |
URL | https://echa.europa.eu/substance-information/-/substanceinfo/100.007.588 | |
Description | The European Chemicals Agency (ECHA) is an agency of the European Union which is the driving force among regulatory authorities in implementing the EU's groundbreaking chemicals legislation for the benefit of human health and the environment as well as for innovation and competitiveness. | |
Explanation | Use of the information, documents and data from the ECHA website is subject to the terms and conditions of this Legal Notice, and subject to other binding limitations provided for under applicable law, the information, documents and data made available on the ECHA website may be reproduced, distributed and/or used, totally or in part, for non-commercial purposes provided that ECHA is acknowledged as the source: "Source: European Chemicals Agency, http://echa.europa.eu/". Such acknowledgement must be included in each copy of the material. ECHA permits and encourages organisations and individuals to create links to the ECHA website under the following cumulative conditions: Links can only be made to webpages that provide a link to the Legal Notice page. | |
Retrosynthesis Analysis
AI-Powered Synthesis Planning: Our tool employs the Template_relevance Pistachio, Template_relevance Bkms_metabolic, Template_relevance Pistachio_ringbreaker, Template_relevance Reaxys, Template_relevance Reaxys_biocatalysis model, leveraging a vast database of chemical reactions to predict feasible synthetic routes.
One-Step Synthesis Focus: Specifically designed for one-step synthesis, it provides concise and direct routes for your target compounds, streamlining the synthesis process.
Accurate Predictions: Utilizing the extensive PISTACHIO, BKMS_METABOLIC, PISTACHIO_RINGBREAKER, REAXYS, REAXYS_BIOCATALYSIS database, our tool offers high-accuracy predictions, reflecting the latest in chemical research and data.
Strategy Settings
Precursor scoring | Relevance Heuristic |
---|---|
Min. plausibility | 0.01 |
Model | Template_relevance |
Template Set | Pistachio/Bkms_metabolic/Pistachio_ringbreaker/Reaxys/Reaxys_biocatalysis |
Top-N result to add to graph | 6 |
Feasible Synthetic Routes
Q & A
ANone: Cyanine dyes interact with their targets through various mechanisms, including:
- Intercalation: Cyanine dyes can insert themselves between the base pairs of DNA, altering its structure and function. This interaction is often accompanied by significant changes in the dye's optical properties, making them valuable probes for DNA detection. []
- Aggregation: Cyanine dyes readily self-assemble into aggregates with distinct spectral properties. This aggregation can be influenced by factors like concentration, solvent, and the presence of other molecules like surfactants or biomolecules. [, ]
- Electrostatic Interactions: The positively charged nature of many cyanine dyes facilitates their interaction with negatively charged molecules like DNA, cell membranes, and proteins. [, ]
- Covalent Binding: Cyanine dyes can be functionalized with reactive groups to enable covalent attachment to biomolecules, creating targeted fluorescent probes. [, ]
- Fluorescence Quenching/Enhancement: Binding to targets can alter the fluorescence intensity of cyanine dyes, enabling their use as sensors. []
- Photothermal Conversion: H-aggregates of certain cyanine dyes exhibit enhanced photothermal conversion efficiency, making them promising for photothermal therapy. []
- Reactive Oxygen Species (ROS) Generation: Some cyanine dye aggregates can generate ROS upon light irradiation, contributing to their phototoxic effects. []
- Photocleavage of DNA: Certain cyanine dyes can induce DNA strand breaks upon light activation, highlighting their potential for photodynamic therapy. []
ANone: Cyanine dyes share a common structural motif consisting of two heterocyclic nitrogen-containing rings linked by a polymethine chain with an odd number of carbons. Their general structure can be represented as:
- NMR Spectroscopy: Provides valuable information about the structure and conformation of cyanine dyes. For instance, the chemical shifts of the polymethine chain carbons in merocyanines are sensitive to the ground-state polarization. []
ANone:
- Solvent Dependence: The spectral properties of cyanine dyes are heavily influenced by the solvent polarity. For example, merocyanines exhibit positive solvatochromism, meaning their absorption spectra shift to longer wavelengths with increasing solvent polarity. []
- Aggregation Effects: Aggregation of cyanine dyes can lead to changes in their absorption and emission spectra, fluorescence quantum yield, and photostability. [, , ]
- Fluorescent probes: Their sensitivity to environmental changes makes them useful as probes for pH, polarity, and viscosity. [, ]
- Bioimaging: Cyanine dyes are widely used in bioimaging due to their ability to label biomolecules and their emission in the NIR region, which offers deeper tissue penetration and reduced background autofluorescence. [, , ]
- Photodynamic Therapy: Some cyanine dyes generate ROS upon light irradiation, leading to cell death and making them potential candidates for photodynamic therapy. []
- Dye-Sensitized Solar Cells: Cyanine dyes can be used as sensitizers in solar cells due to their strong absorption in the visible region and ability to inject electrons into semiconductors like TiO2. []
ANone: Computational chemistry plays a crucial role in understanding and predicting the properties of cyanine dyes:
- Molecular Dynamics Simulations: These simulations help unravel the self-assembly behavior of cyanine dyes in solution, revealing the driving forces behind aggregate formation and morphology. []
- Quantum Chemical Calculations: Quantum chemical calculations are employed to predict various properties of cyanine dyes, including:
- Electronic Spectra: Calculating the electronic transitions and excited state energies helps explain their absorption and emission characteristics. [, , ]
- Nonlinear Optical Properties: Theoretical studies can predict the nonlinear optical properties of cyanine dyes, which are crucial for applications like optical limiting and frequency conversion. []
- Structure-Property Relationships: Computational methods enable the exploration of how structural modifications impact the electronic properties and reactivity of cyanine dyes. [, ]
ANone: The structure of cyanine dyes profoundly impacts their properties and activities. Key SAR trends include:
- Polymethine Chain Length: Increasing the number of vinylene units in the polymethine chain leads to a bathochromic shift in absorption and emission, moving them further into the NIR region. [, ]
- Heterocyclic Ring Modifications: The choice of heterocyclic rings significantly influences the dye's absorption wavelength, fluorescence quantum yield, and stability. [, , ]
- Substituents: Introducing electron-donating or electron-withdrawing groups on the heterocyclic rings or the polymethine chain can fine-tune the dye's absorption and emission properties, as well as its solubility and aggregation behavior. [, , ]
- Charge and Counterions: The nature of the counterion can impact the aggregation behavior and solubility of cyanine dyes. For instance, the presence of specific counterions can promote J-aggregate formation. [, ]
ANone:
- Stability Challenges: Cyanine dyes can be susceptible to degradation under certain conditions, such as exposure to light, heat, oxidizing agents, and extremes of pH. []
- Formulation Strategies: Various strategies are employed to enhance their stability and optimize their performance:
- Encapsulation: Encapsulating cyanine dyes within nanoparticles or other protective matrices can shield them from degradation and improve their biocompatibility. [, ]
- Structural Modifications: Introducing sterically hindering groups or modifying the polymethine chain can enhance their photostability and resistance to aggregation. [, ]
- Additives: Adding antioxidants or other stabilizers to formulations can help prevent degradation. []
Disclaimer and Information on In-Vitro Research Products
Please be aware that all articles and product information presented on BenchChem are intended solely for informational purposes. The products available for purchase on BenchChem are specifically designed for in-vitro studies, which are conducted outside of living organisms. In-vitro studies, derived from the Latin term "in glass," involve experiments performed in controlled laboratory settings using cells or tissues. It is important to note that these products are not categorized as medicines or drugs, and they have not received approval from the FDA for the prevention, treatment, or cure of any medical condition, ailment, or disease. We must emphasize that any form of bodily introduction of these products into humans or animals is strictly prohibited by law. It is essential to adhere to these guidelines to ensure compliance with legal and ethical standards in research and experimentation.