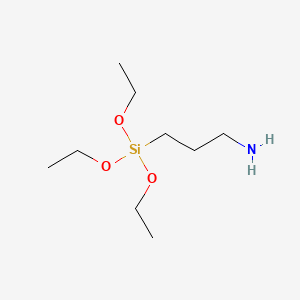
3-Aminopropyltriethoxysilane
Overview
Description
3-Aminopropyltriethoxysilane is an organosilane compound with the chemical formula C₉H₂₃NO₃Si. It is frequently used in the process of silanization, which involves the functionalization of surfaces with alkoxysilane molecules. This compound is particularly valuable for its ability to covalently attach organic films to metal oxides such as silica and titania .
Mechanism of Action
Target of Action
The primary targets of APTES are surfaces of various materials, particularly metal oxide nanoparticles (MONPs) such as silica and titania . APTES is frequently used in the process of silanization, which involves the functionalization of these surfaces with alkoxysilane molecules .
Mode of Action
APTES interacts with its targets through a process known as silanization. This process involves the covalent attachment of organic films to metal oxides . The introduction of an amine group (NH2) enhances the dispersibility and anti-bacterial property of the treated surfaces . APTES can also be used to covalently bond thermoplastics to poly(dimethylsiloxane) (PDMS) .
Biochemical Pathways
The biochemical pathways affected by APTES primarily involve the modification of metal oxide nanoparticle surfaces. The introduction of the amine group enhances their dispersibility and anti-bacterial property . This modification provides a linkage for numerous organic, inorganic, or biochemical attachments that are essential to various applications such as drug delivery, contaminants removal, catalyst immobilization, and medical imaging .
Pharmacokinetics
Its fumes are destructive to the mucous membranes and the upper respiratory tract .
Result of Action
The result of APTES action is the creation of functionalized surfaces with enhanced properties. These surfaces have improved dispersibility, anti-bacterial properties , and can form stable covalent bonds with other materials . This makes APTES a valuable tool in the synthesis of materials and in various applications such as biosensors .
Action Environment
The action of APTES can be influenced by environmental factors. For instance, the process of silanization requires specific conditions for optimal results . Furthermore, APTES should be used in a controlled environment (e.g., a fume hood) due to its toxicity .
Biochemical Analysis
Biochemical Properties
3-Aminopropyltriethoxysilane has been extensively used as a silanization agent for the chemical modification of metal oxide nanoparticle surfaces . It provides a linkage for numerous organic, inorganic, or biochemical attachments that are essential to various applications .
Cellular Effects
This compound-functionalized surfaces have been shown to be non-toxic to embryonic rat cardiomyocytes in vitro . Further experimentation is needed to evaluate toxicity to other cell types in extended culture .
Molecular Mechanism
The molecular mechanism of this compound involves the initial hydroxylation of silicon dioxide or glass with subsequent hydrolysis of the this compound ethoxy groups with ethanol as the leaving group, resulting in an aminopropyl-terminated surface . The type of solvent strongly affects the density and conformation of the covalently attached this compound layer .
Preparation Methods
Synthetic Routes and Reaction Conditions
3-Aminopropyltriethoxysilane can be synthesized through the reaction of 3-aminopropylamine with triethoxysilane. The reaction typically occurs under anhydrous conditions to prevent hydrolysis of the ethoxy groups. The general reaction is as follows:
3-Aminopropylamine+Triethoxysilane→this compound+By-products
Industrial Production Methods
In industrial settings, this compound is produced using large-scale reactors where the reaction conditions are carefully controlled to optimize yield and purity. The process involves the use of catalysts to accelerate the reaction and ensure complete conversion of the starting materials .
Chemical Reactions Analysis
Types of Reactions
3-Aminopropyltriethoxysilane undergoes several types of chemical reactions, including:
Hydrolysis: The ethoxy groups can be hydrolyzed to form silanol groups.
Condensation: The silanol groups can condense to form siloxane bonds.
Substitution: The amino group can participate in nucleophilic substitution reactions.
Common Reagents and Conditions
Hydrolysis: Typically performed in the presence of water or moisture.
Condensation: Often catalyzed by acids or bases.
Substitution: Commonly involves reagents such as alkyl halides or acyl chlorides.
Major Products
Hydrolysis: Produces silanol derivatives.
Condensation: Forms siloxane networks.
Substitution: Yields various substituted amines.
Scientific Research Applications
3-Aminopropyltriethoxysilane has a wide range of applications in scientific research:
Chemistry: Used as a silanization agent for modifying surfaces of metal oxides and other materials.
Biology: Employed in the functionalization of surfaces for cell culture and biosensing applications.
Medicine: Utilized in the preparation of drug delivery systems and medical imaging agents.
Industry: Applied in the production of adhesives, sealants, and coatings.
Comparison with Similar Compounds
3-Aminopropyltriethoxysilane is often compared with other organosilanes such as:
3-Aminopropyltrimethoxysilane: Similar in structure but with methoxy groups instead of ethoxy groups.
N-(2-Aminoethyl)-3-aminopropyltriethoxysilane: Contains an additional amino group, providing more sites for functionalization.
3-Mercaptopropyltrimethoxysilane: Contains a thiol group instead of an amino group, offering different reactivity.
The uniqueness of this compound lies in its balance of reactivity and stability, making it suitable for a wide range of applications .
Properties
IUPAC Name |
3-triethoxysilylpropan-1-amine | |
---|---|---|
Source | PubChem | |
URL | https://pubchem.ncbi.nlm.nih.gov | |
Description | Data deposited in or computed by PubChem | |
InChI |
InChI=1S/C9H23NO3Si/c1-4-11-14(12-5-2,13-6-3)9-7-8-10/h4-10H2,1-3H3 | |
Source | PubChem | |
URL | https://pubchem.ncbi.nlm.nih.gov | |
Description | Data deposited in or computed by PubChem | |
InChI Key |
WYTZZXDRDKSJID-UHFFFAOYSA-N | |
Source | PubChem | |
URL | https://pubchem.ncbi.nlm.nih.gov | |
Description | Data deposited in or computed by PubChem | |
Canonical SMILES |
CCO[Si](CCCN)(OCC)OCC | |
Source | PubChem | |
URL | https://pubchem.ncbi.nlm.nih.gov | |
Description | Data deposited in or computed by PubChem | |
Molecular Formula |
C9H23NO3Si | |
Source | PubChem | |
URL | https://pubchem.ncbi.nlm.nih.gov | |
Description | Data deposited in or computed by PubChem | |
Related CAS |
29159-37-3 | |
Record name | γ-Aminopropyltriethoxysilane homopolymer | |
Source | CAS Common Chemistry | |
URL | https://commonchemistry.cas.org/detail?cas_rn=29159-37-3 | |
Description | CAS Common Chemistry is an open community resource for accessing chemical information. Nearly 500,000 chemical substances from CAS REGISTRY cover areas of community interest, including common and frequently regulated chemicals, and those relevant to high school and undergraduate chemistry classes. This chemical information, curated by our expert scientists, is provided in alignment with our mission as a division of the American Chemical Society. | |
Explanation | The data from CAS Common Chemistry is provided under a CC-BY-NC 4.0 license, unless otherwise stated. | |
DSSTOX Substance ID |
DTXSID2027333 | |
Record name | 3-Aminopropyltriethoxysilane | |
Source | EPA DSSTox | |
URL | https://comptox.epa.gov/dashboard/DTXSID2027333 | |
Description | DSSTox provides a high quality public chemistry resource for supporting improved predictive toxicology. | |
Molecular Weight |
221.37 g/mol | |
Source | PubChem | |
URL | https://pubchem.ncbi.nlm.nih.gov | |
Description | Data deposited in or computed by PubChem | |
Physical Description |
Liquid, Liquid; [HSDB] Colorless or light yellow liquid with a fishy odor; [CHEMINFO] Clear colorless liquid; [MSDSonline] | |
Record name | 1-Propanamine, 3-(triethoxysilyl)- | |
Source | EPA Chemicals under the TSCA | |
URL | https://www.epa.gov/chemicals-under-tsca | |
Description | EPA Chemicals under the Toxic Substances Control Act (TSCA) collection contains information on chemicals and their regulations under TSCA, including non-confidential content from the TSCA Chemical Substance Inventory and Chemical Data Reporting. | |
Record name | 3-(Triethoxysilyl)propylamine | |
Source | Haz-Map, Information on Hazardous Chemicals and Occupational Diseases | |
URL | https://haz-map.com/Agents/7560 | |
Description | Haz-Map® is an occupational health database designed for health and safety professionals and for consumers seeking information about the adverse effects of workplace exposures to chemical and biological agents. | |
Explanation | Copyright (c) 2022 Haz-Map(R). All rights reserved. Unless otherwise indicated, all materials from Haz-Map are copyrighted by Haz-Map(R). No part of these materials, either text or image may be used for any purpose other than for personal use. Therefore, reproduction, modification, storage in a retrieval system or retransmission, in any form or by any means, electronic, mechanical or otherwise, for reasons other than personal use, is strictly prohibited without prior written permission. | |
Boiling Point |
217 °C | |
Record name | 3-(Triethoxysilyl)propylamine | |
Source | Hazardous Substances Data Bank (HSDB) | |
URL | https://pubchem.ncbi.nlm.nih.gov/source/hsdb/5767 | |
Description | The Hazardous Substances Data Bank (HSDB) is a toxicology database that focuses on the toxicology of potentially hazardous chemicals. It provides information on human exposure, industrial hygiene, emergency handling procedures, environmental fate, regulatory requirements, nanomaterials, and related areas. The information in HSDB has been assessed by a Scientific Review Panel. | |
Flash Point |
98 °C (closed cup) | |
Record name | 3-(Triethoxysilyl)propylamine | |
Source | Hazardous Substances Data Bank (HSDB) | |
URL | https://pubchem.ncbi.nlm.nih.gov/source/hsdb/5767 | |
Description | The Hazardous Substances Data Bank (HSDB) is a toxicology database that focuses on the toxicology of potentially hazardous chemicals. It provides information on human exposure, industrial hygiene, emergency handling procedures, environmental fate, regulatory requirements, nanomaterials, and related areas. The information in HSDB has been assessed by a Scientific Review Panel. | |
Density |
0.94 at 25 °C, Density = 0.9506 g/cu m at 20 °C | |
Record name | 3-(Triethoxysilyl)propylamine | |
Source | Hazardous Substances Data Bank (HSDB) | |
URL | https://pubchem.ncbi.nlm.nih.gov/source/hsdb/5767 | |
Description | The Hazardous Substances Data Bank (HSDB) is a toxicology database that focuses on the toxicology of potentially hazardous chemicals. It provides information on human exposure, industrial hygiene, emergency handling procedures, environmental fate, regulatory requirements, nanomaterials, and related areas. The information in HSDB has been assessed by a Scientific Review Panel. | |
Vapor Pressure |
0.01 [mmHg], 3.3 kPa at 121 °C extrapolated to 2 Pa at 20 °C (0.015 mm Hg) | |
Record name | 3-(Triethoxysilyl)propylamine | |
Source | Haz-Map, Information on Hazardous Chemicals and Occupational Diseases | |
URL | https://haz-map.com/Agents/7560 | |
Description | Haz-Map® is an occupational health database designed for health and safety professionals and for consumers seeking information about the adverse effects of workplace exposures to chemical and biological agents. | |
Explanation | Copyright (c) 2022 Haz-Map(R). All rights reserved. Unless otherwise indicated, all materials from Haz-Map are copyrighted by Haz-Map(R). No part of these materials, either text or image may be used for any purpose other than for personal use. Therefore, reproduction, modification, storage in a retrieval system or retransmission, in any form or by any means, electronic, mechanical or otherwise, for reasons other than personal use, is strictly prohibited without prior written permission. | |
Record name | 3-(Triethoxysilyl)propylamine | |
Source | Hazardous Substances Data Bank (HSDB) | |
URL | https://pubchem.ncbi.nlm.nih.gov/source/hsdb/5767 | |
Description | The Hazardous Substances Data Bank (HSDB) is a toxicology database that focuses on the toxicology of potentially hazardous chemicals. It provides information on human exposure, industrial hygiene, emergency handling procedures, environmental fate, regulatory requirements, nanomaterials, and related areas. The information in HSDB has been assessed by a Scientific Review Panel. | |
Impurities |
Ethyl alcohol (0-1%), 2-butanone (0-2%), dibenzoyl peroxide (0-1%) (purity 98% - 100%)[Organization for Economic Cooperation and Development; Screening Information Data Set for 3-Aminopropyltriethoxysilane | |
Record name | 3-(Triethoxysilyl)propylamine | |
Source | Hazardous Substances Data Bank (HSDB) | |
URL | https://pubchem.ncbi.nlm.nih.gov/source/hsdb/5767 | |
Description | The Hazardous Substances Data Bank (HSDB) is a toxicology database that focuses on the toxicology of potentially hazardous chemicals. It provides information on human exposure, industrial hygiene, emergency handling procedures, environmental fate, regulatory requirements, nanomaterials, and related areas. The information in HSDB has been assessed by a Scientific Review Panel. | |
Color/Form |
Liquid | |
CAS No. |
919-30-2 | |
Record name | (3-Aminopropyl)triethoxysilane | |
Source | CAS Common Chemistry | |
URL | https://commonchemistry.cas.org/detail?cas_rn=919-30-2 | |
Description | CAS Common Chemistry is an open community resource for accessing chemical information. Nearly 500,000 chemical substances from CAS REGISTRY cover areas of community interest, including common and frequently regulated chemicals, and those relevant to high school and undergraduate chemistry classes. This chemical information, curated by our expert scientists, is provided in alignment with our mission as a division of the American Chemical Society. | |
Explanation | The data from CAS Common Chemistry is provided under a CC-BY-NC 4.0 license, unless otherwise stated. | |
Record name | 3-(Triethoxysilyl)propylamine | |
Source | ChemIDplus | |
URL | https://pubchem.ncbi.nlm.nih.gov/substance/?source=chemidplus&sourceid=0000919302 | |
Description | ChemIDplus is a free, web search system that provides access to the structure and nomenclature authority files used for the identification of chemical substances cited in National Library of Medicine (NLM) databases, including the TOXNET system. | |
Record name | 3-Aminopropyltriethoxysilane | |
Source | DTP/NCI | |
URL | https://dtp.cancer.gov/dtpstandard/servlet/dwindex?searchtype=NSC&outputformat=html&searchlist=95428 | |
Description | The NCI Development Therapeutics Program (DTP) provides services and resources to the academic and private-sector research communities worldwide to facilitate the discovery and development of new cancer therapeutic agents. | |
Explanation | Unless otherwise indicated, all text within NCI products is free of copyright and may be reused without our permission. Credit the National Cancer Institute as the source. | |
Record name | 1-Propanamine, 3-(triethoxysilyl)- | |
Source | EPA Chemicals under the TSCA | |
URL | https://www.epa.gov/chemicals-under-tsca | |
Description | EPA Chemicals under the Toxic Substances Control Act (TSCA) collection contains information on chemicals and their regulations under TSCA, including non-confidential content from the TSCA Chemical Substance Inventory and Chemical Data Reporting. | |
Record name | 3-Aminopropyltriethoxysilane | |
Source | EPA DSSTox | |
URL | https://comptox.epa.gov/dashboard/DTXSID2027333 | |
Description | DSSTox provides a high quality public chemistry resource for supporting improved predictive toxicology. | |
Record name | 3-aminopropyltriethoxysilane | |
Source | European Chemicals Agency (ECHA) | |
URL | https://echa.europa.eu/substance-information/-/substanceinfo/100.011.863 | |
Description | The European Chemicals Agency (ECHA) is an agency of the European Union which is the driving force among regulatory authorities in implementing the EU's groundbreaking chemicals legislation for the benefit of human health and the environment as well as for innovation and competitiveness. | |
Explanation | Use of the information, documents and data from the ECHA website is subject to the terms and conditions of this Legal Notice, and subject to other binding limitations provided for under applicable law, the information, documents and data made available on the ECHA website may be reproduced, distributed and/or used, totally or in part, for non-commercial purposes provided that ECHA is acknowledged as the source: "Source: European Chemicals Agency, http://echa.europa.eu/". Such acknowledgement must be included in each copy of the material. ECHA permits and encourages organisations and individuals to create links to the ECHA website under the following cumulative conditions: Links can only be made to webpages that provide a link to the Legal Notice page. | |
Record name | 3-(TRIETHOXYSILYL)PROPYLAMINE | |
Source | FDA Global Substance Registration System (GSRS) | |
URL | https://gsrs.ncats.nih.gov/ginas/app/beta/substances/L8S6UBW552 | |
Description | The FDA Global Substance Registration System (GSRS) enables the efficient and accurate exchange of information on what substances are in regulated products. Instead of relying on names, which vary across regulatory domains, countries, and regions, the GSRS knowledge base makes it possible for substances to be defined by standardized, scientific descriptions. | |
Explanation | Unless otherwise noted, the contents of the FDA website (www.fda.gov), both text and graphics, are not copyrighted. They are in the public domain and may be republished, reprinted and otherwise used freely by anyone without the need to obtain permission from FDA. Credit to the U.S. Food and Drug Administration as the source is appreciated but not required. | |
Record name | 3-(Triethoxysilyl)propylamine | |
Source | Hazardous Substances Data Bank (HSDB) | |
URL | https://pubchem.ncbi.nlm.nih.gov/source/hsdb/5767 | |
Description | The Hazardous Substances Data Bank (HSDB) is a toxicology database that focuses on the toxicology of potentially hazardous chemicals. It provides information on human exposure, industrial hygiene, emergency handling procedures, environmental fate, regulatory requirements, nanomaterials, and related areas. The information in HSDB has been assessed by a Scientific Review Panel. | |
Melting Point |
-70 °C | |
Record name | 3-(Triethoxysilyl)propylamine | |
Source | Hazardous Substances Data Bank (HSDB) | |
URL | https://pubchem.ncbi.nlm.nih.gov/source/hsdb/5767 | |
Description | The Hazardous Substances Data Bank (HSDB) is a toxicology database that focuses on the toxicology of potentially hazardous chemicals. It provides information on human exposure, industrial hygiene, emergency handling procedures, environmental fate, regulatory requirements, nanomaterials, and related areas. The information in HSDB has been assessed by a Scientific Review Panel. | |
Synthesis routes and methods I
Procedure details
Synthesis routes and methods II
Procedure details
Retrosynthesis Analysis
AI-Powered Synthesis Planning: Our tool employs the Template_relevance Pistachio, Template_relevance Bkms_metabolic, Template_relevance Pistachio_ringbreaker, Template_relevance Reaxys, Template_relevance Reaxys_biocatalysis model, leveraging a vast database of chemical reactions to predict feasible synthetic routes.
One-Step Synthesis Focus: Specifically designed for one-step synthesis, it provides concise and direct routes for your target compounds, streamlining the synthesis process.
Accurate Predictions: Utilizing the extensive PISTACHIO, BKMS_METABOLIC, PISTACHIO_RINGBREAKER, REAXYS, REAXYS_BIOCATALYSIS database, our tool offers high-accuracy predictions, reflecting the latest in chemical research and data.
Strategy Settings
Precursor scoring | Relevance Heuristic |
---|---|
Min. plausibility | 0.01 |
Model | Template_relevance |
Template Set | Pistachio/Bkms_metabolic/Pistachio_ringbreaker/Reaxys/Reaxys_biocatalysis |
Top-N result to add to graph | 6 |
Feasible Synthetic Routes
ANone: 3-Aminopropyltriethoxysilane (APTES) primarily interacts with target surfaces through its silane group (Si(OC₂H₅)₃). The ethoxy groups (OC₂H₅) of APTES undergo hydrolysis in the presence of water, forming silanol groups (Si-OH). These silanol groups can then condense with hydroxyl groups (OH) present on various surfaces, such as silica, glass, metal oxides, and even some polymers, forming strong covalent Si-O-Si bonds. This effectively anchors APTES to the surface.
ANone:
- Spectroscopic Data:
ANone: APTES exhibits compatibility with a variety of materials, including:
- Inorganic materials: Silica [, , , , ], glass [, , ], metal oxides [, ], clays [, , , ], and zeolites [].
- Polymers: Polypropylene [], poly(ethylene terephthalate) [], and others [, , ].
- Thermal stability: The thermal stability of APTES-modified materials depends on the specific application and the presence of other components. In general, APTES itself degrades at temperatures above 200 °C [].
- Solvent effects: APTES films deposited from non-polar solvents exhibit higher density and improved mechanical properties compared to those deposited from polar solvents [].
- Surface modification: APTES is extensively used to modify the surface properties of various materials, such as enhancing adhesion, promoting cell attachment [], and introducing desired functionalities.
- Composite materials: APTES acts as a coupling agent, improving the interfacial adhesion between inorganic fillers and polymer matrices, resulting in enhanced mechanical properties [, ].
- Sensors: APTES can be used to immobilize biomolecules on sensor surfaces for biosensing applications [, ].
ANone: While APTES itself is not a catalyst, the amine group it introduces can act as a catalyst or a support for catalytic species in various reactions:
- Catalyst support: APTES can be used to anchor metal nanoparticles or other catalytic species onto a support material, improving their stability and activity. For example, APTES-modified magnetic nanoparticles were used to immobilize metalloporphyrins, creating efficient and recyclable catalysts for cyclohexane oxidation [].
- Organic synthesis: APTES can be used as a catalyst or catalyst support in various organic reactions, including oxidation, reduction, coupling reactions, and heterocyclic synthesis [].
- Environmental remediation: APTES-modified materials have been studied for the removal of pollutants from wastewater, such as heavy metals and dyes [, ].
Disclaimer and Information on In-Vitro Research Products
Please be aware that all articles and product information presented on BenchChem are intended solely for informational purposes. The products available for purchase on BenchChem are specifically designed for in-vitro studies, which are conducted outside of living organisms. In-vitro studies, derived from the Latin term "in glass," involve experiments performed in controlled laboratory settings using cells or tissues. It is important to note that these products are not categorized as medicines or drugs, and they have not received approval from the FDA for the prevention, treatment, or cure of any medical condition, ailment, or disease. We must emphasize that any form of bodily introduction of these products into humans or animals is strictly prohibited by law. It is essential to adhere to these guidelines to ensure compliance with legal and ethical standards in research and experimentation.