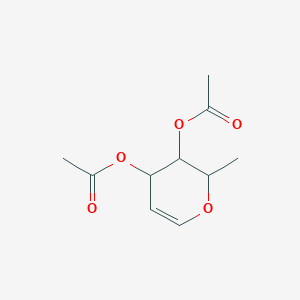
3,4-Di-O-acetyl-6-deoxy-L-glucal
Overview
Description
3,4-Di-O-acetyl-6-deoxy-L-glucal is a chemical compound with the molecular formula C10H14O5. It is a derivative of L-glucal, where the hydroxyl groups at positions 3 and 4 are acetylated, and the hydroxyl group at position 6 is removed. This compound is also known as Di-O-acetyl-L-rhamnal. It is commonly used as an intermediate in the synthesis of various complex molecules, particularly in the field of carbohydrate chemistry.
Preparation Methods
Synthetic Routes and Reaction Conditions
The synthesis of 3,4-Di-O-acetyl-6-deoxy-L-glucal typically involves the acetylation of L-glucal. One common method includes the following steps:
Starting Material: L-glucal.
Acetylation: The hydroxyl groups at positions 3 and 4 of L-glucal are acetylated using acetic anhydride in the presence of a catalyst such as pyridine.
Deoxygenation: The hydroxyl group at position 6 is removed through a reduction reaction, often using reagents like lithium aluminum hydride or other reducing agents.
Industrial Production Methods
Industrial production of this compound follows similar synthetic routes but on a larger scale. The process involves:
Bulk Acetylation: Large quantities of L-glucal are acetylated using acetic anhydride and a suitable catalyst.
Purification: The product is purified through distillation or recrystallization to achieve the desired purity level.
Quality Control: The final product undergoes rigorous quality control to ensure it meets industrial standards.
Chemical Reactions Analysis
Types of Reactions
3,4-Di-O-acetyl-6-deoxy-L-glucal undergoes various chemical reactions, including:
Oxidation: The compound can be oxidized to form corresponding acids or aldehydes.
Reduction: Reduction reactions can further deoxygenate the molecule or modify the acetyl groups.
Substitution: The acetyl groups can be substituted with other functional groups through nucleophilic substitution reactions.
Common Reagents and Conditions
Oxidation: Common oxidizing agents include potassium permanganate and chromium trioxide.
Reduction: Reducing agents such as lithium aluminum hydride or sodium borohydride are frequently used.
Substitution: Nucleophiles like amines or alcohols can be used to substitute the acetyl groups under basic conditions.
Major Products
Oxidation Products: Corresponding acids or aldehydes.
Reduction Products: Deoxygenated derivatives or modified acetyl groups.
Substitution Products: Compounds with new functional groups replacing the acetyl groups.
Scientific Research Applications
3,4-Di-O-acetyl-6-deoxy-L-glucal has several applications in scientific research:
Chemistry: It is used as an intermediate in the synthesis of complex carbohydrates and glycosides.
Biology: The compound is utilized in the study of carbohydrate metabolism and enzyme interactions.
Medicine: It serves as a precursor in the synthesis of pharmaceutical compounds, particularly those involving glycosylation.
Industry: The compound is used in the production of specialty chemicals and as a building block for various industrial processes.
Mechanism of Action
The mechanism of action of 3,4-Di-O-acetyl-6-deoxy-L-glucal involves its role as an intermediate in chemical reactions. The acetyl groups at positions 3 and 4 make the molecule more reactive, facilitating various chemical transformations. The compound can interact with enzymes and other biological molecules, influencing metabolic pathways and biochemical processes.
Comparison with Similar Compounds
Similar Compounds
Tri-O-acetyl-D-glucal: Similar in structure but with an additional acetyl group.
D-Glucal: The non-acetylated form of the compound.
Tri-O-benzyl-D-glucal: Similar structure with benzyl groups instead of acetyl groups.
Uniqueness
3,4-Di-O-acetyl-6-deoxy-L-glucal is unique due to its specific acetylation pattern and the absence of the hydroxyl group at position 6. This unique structure makes it a valuable intermediate in the synthesis of various complex molecules, particularly in carbohydrate chemistry.
Properties
IUPAC Name |
(3-acetyloxy-2-methyl-3,4-dihydro-2H-pyran-4-yl) acetate | |
---|---|---|
Details | Computed by LexiChem 2.6.6 (PubChem release 2019.06.18) | |
Source | PubChem | |
URL | https://pubchem.ncbi.nlm.nih.gov | |
Description | Data deposited in or computed by PubChem | |
InChI |
InChI=1S/C10H14O5/c1-6-10(15-8(3)12)9(4-5-13-6)14-7(2)11/h4-6,9-10H,1-3H3 | |
Details | Computed by InChI 1.0.5 (PubChem release 2019.06.18) | |
Source | PubChem | |
URL | https://pubchem.ncbi.nlm.nih.gov | |
Description | Data deposited in or computed by PubChem | |
InChI Key |
NDEGMKQAZZBNBB-UHFFFAOYSA-N | |
Details | Computed by InChI 1.0.5 (PubChem release 2019.06.18) | |
Source | PubChem | |
URL | https://pubchem.ncbi.nlm.nih.gov | |
Description | Data deposited in or computed by PubChem | |
Canonical SMILES |
CC1C(C(C=CO1)OC(=O)C)OC(=O)C | |
Details | Computed by OEChem 2.1.5 (PubChem release 2019.06.18) | |
Source | PubChem | |
URL | https://pubchem.ncbi.nlm.nih.gov | |
Description | Data deposited in or computed by PubChem | |
Molecular Formula |
C10H14O5 | |
Details | Computed by PubChem 2.1 (PubChem release 2019.06.18) | |
Source | PubChem | |
URL | https://pubchem.ncbi.nlm.nih.gov | |
Description | Data deposited in or computed by PubChem | |
DSSTOX Substance ID |
DTXSID80956298 | |
Record name | 3,4-Di-O-acetyl-2,6-anhydro-1,5-dideoxyhex-5-enitol | |
Source | EPA DSSTox | |
URL | https://comptox.epa.gov/dashboard/DTXSID80956298 | |
Description | DSSTox provides a high quality public chemistry resource for supporting improved predictive toxicology. | |
Molecular Weight |
214.21 g/mol | |
Details | Computed by PubChem 2.1 (PubChem release 2021.05.07) | |
Source | PubChem | |
URL | https://pubchem.ncbi.nlm.nih.gov | |
Description | Data deposited in or computed by PubChem | |
CAS No. |
34819-86-8 | |
Record name | 1,5-Dianhydro-2,6-dideoxy-L-arabino-hex-1-enitol diacetate | |
Source | ChemIDplus | |
URL | https://pubchem.ncbi.nlm.nih.gov/substance/?source=chemidplus&sourceid=0034819868 | |
Description | ChemIDplus is a free, web search system that provides access to the structure and nomenclature authority files used for the identification of chemical substances cited in National Library of Medicine (NLM) databases, including the TOXNET system. | |
Record name | 3,4-Di-O-acetyl-2,6-anhydro-1,5-dideoxyhex-5-enitol | |
Source | EPA DSSTox | |
URL | https://comptox.epa.gov/dashboard/DTXSID80956298 | |
Description | DSSTox provides a high quality public chemistry resource for supporting improved predictive toxicology. | |
Record name | 1,5-dianhydro-2,6-dideoxy-L-arabino-hex-1-enitol diacetate | |
Source | European Chemicals Agency (ECHA) | |
URL | https://echa.europa.eu/substance-information/-/substanceinfo/100.047.465 | |
Description | The European Chemicals Agency (ECHA) is an agency of the European Union which is the driving force among regulatory authorities in implementing the EU's groundbreaking chemicals legislation for the benefit of human health and the environment as well as for innovation and competitiveness. | |
Explanation | Use of the information, documents and data from the ECHA website is subject to the terms and conditions of this Legal Notice, and subject to other binding limitations provided for under applicable law, the information, documents and data made available on the ECHA website may be reproduced, distributed and/or used, totally or in part, for non-commercial purposes provided that ECHA is acknowledged as the source: "Source: European Chemicals Agency, http://echa.europa.eu/". Such acknowledgement must be included in each copy of the material. ECHA permits and encourages organisations and individuals to create links to the ECHA website under the following cumulative conditions: Links can only be made to webpages that provide a link to the Legal Notice page. | |
Retrosynthesis Analysis
AI-Powered Synthesis Planning: Our tool employs the Template_relevance Pistachio, Template_relevance Bkms_metabolic, Template_relevance Pistachio_ringbreaker, Template_relevance Reaxys, Template_relevance Reaxys_biocatalysis model, leveraging a vast database of chemical reactions to predict feasible synthetic routes.
One-Step Synthesis Focus: Specifically designed for one-step synthesis, it provides concise and direct routes for your target compounds, streamlining the synthesis process.
Accurate Predictions: Utilizing the extensive PISTACHIO, BKMS_METABOLIC, PISTACHIO_RINGBREAKER, REAXYS, REAXYS_BIOCATALYSIS database, our tool offers high-accuracy predictions, reflecting the latest in chemical research and data.
Strategy Settings
Precursor scoring | Relevance Heuristic |
---|---|
Min. plausibility | 0.01 |
Model | Template_relevance |
Template Set | Pistachio/Bkms_metabolic/Pistachio_ringbreaker/Reaxys/Reaxys_biocatalysis |
Top-N result to add to graph | 6 |
Feasible Synthetic Routes
Disclaimer and Information on In-Vitro Research Products
Please be aware that all articles and product information presented on BenchChem are intended solely for informational purposes. The products available for purchase on BenchChem are specifically designed for in-vitro studies, which are conducted outside of living organisms. In-vitro studies, derived from the Latin term "in glass," involve experiments performed in controlled laboratory settings using cells or tissues. It is important to note that these products are not categorized as medicines or drugs, and they have not received approval from the FDA for the prevention, treatment, or cure of any medical condition, ailment, or disease. We must emphasize that any form of bodily introduction of these products into humans or animals is strictly prohibited by law. It is essential to adhere to these guidelines to ensure compliance with legal and ethical standards in research and experimentation.