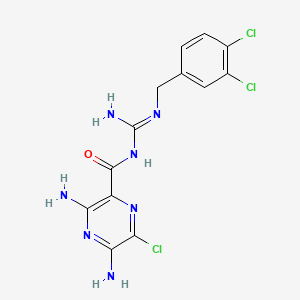
3',4'-Dichlorobenzamil
Overview
Description
3’,4’-Dichlorobenzamil is a synthetic organic compound known for its inhibitory effects on sodium-calcium exchangers, sodium transport, and sarcoplasmic reticulum calcium release channels . It is commonly used in biochemical and physiological research due to its ability to modulate ion transport mechanisms.
Mechanism of Action
Target of Action
The primary target of 3’,4’-Dichlorobenzamil is the sodium-calcium exchanger, sodium transport, and sarcoplasmic reticulum calcium release channels . These targets play a crucial role in maintaining the balance of sodium and calcium ions in cells, which is essential for various cellular functions including muscle contraction and nerve impulse transmission .
Mode of Action
3’,4’-Dichlorobenzamil interacts with its targets by inhibiting the sodium-calcium exchanger and sodium transport . This inhibition disrupts the balance of sodium and calcium ions in cells, leading to changes in cellular functions .
Biochemical Pathways
The inhibition of the sodium-calcium exchanger and sodium transport by 3’,4’-Dichlorobenzamil affects several biochemical pathways. These include the regulation of intracellular calcium levels and the modulation of sodium-dependent transport processes . The downstream effects of these changes can impact various cellular functions, including muscle contraction and nerve impulse transmission .
Pharmacokinetics
It is known that 3’,4’-dichlorobenzamil is soluble in dmso and insoluble in water , which may influence its bioavailability and distribution within the body.
Result of Action
The molecular and cellular effects of 3’,4’-Dichlorobenzamil’s action include changes in intracellular calcium and sodium levels due to the inhibition of the sodium-calcium exchanger and sodium transport . These changes can affect various cellular functions, including muscle contraction and nerve impulse transmission .
Action Environment
The action, efficacy, and stability of 3’,4’-Dichlorobenzamil can be influenced by various environmental factors. For example, the pH and ion concentration of the cellular environment can affect the activity of the sodium-calcium exchanger and sodium transport, and thus the action of 3’,4’-Dichlorobenzamil . Furthermore, the compound’s solubility in different solvents can influence its distribution within the body and its bioavailability .
Biochemical Analysis
Biochemical Properties
3’,4’-Dichlorobenzamil is known to inhibit the sodium-calcium exchanger, a key player in maintaining cellular homeostasis . This interaction affects the balance of sodium and calcium ions within the cell, which can influence various biochemical reactions .
Cellular Effects
In cellular studies, 3’,4’-Dichlorobenzamil has been shown to completely abolish the excystation of Acanthamoeba, a type of amoeba . This suggests that 3’,4’-Dichlorobenzamil can influence cellular processes and functions .
Molecular Mechanism
The molecular mechanism of 3’,4’-Dichlorobenzamil involves its binding to the sodium-calcium exchanger, inhibiting its function . This can lead to changes in ion concentrations within the cell, affecting various cellular processes .
Temporal Effects in Laboratory Settings
In laboratory settings, the effects of 3’,4’-Dichlorobenzamil have been observed over time. For instance, it has been shown to inhibit the excystation of Acanthamoeba, suggesting that it can have long-term effects on cellular function .
Metabolic Pathways
Given its role in inhibiting the sodium-calcium exchanger, it may influence pathways related to ion transport and homeostasis .
Subcellular Localization
Given its role in inhibiting the sodium-calcium exchanger, it is likely to be found in areas of the cell where this exchanger is present .
Preparation Methods
Synthetic Routes and Reaction Conditions: The synthesis of 3’,4’-Dichlorobenzamil involves the reaction of 3,4-dichlorobenzylamine with pyrazine-2-carboxamide under specific conditions. The reaction typically requires a solvent such as dimethyl sulfoxide (DMSO) and a catalyst to facilitate the formation of the desired product .
Industrial Production Methods: Industrial production of 3’,4’-Dichlorobenzamil follows similar synthetic routes but on a larger scale. The process involves optimizing reaction conditions to maximize yield and purity. The compound is then purified using techniques such as recrystallization or chromatography to achieve the desired quality .
Chemical Reactions Analysis
Types of Reactions: 3’,4’-Dichlorobenzamil undergoes various chemical reactions, including:
Substitution Reactions: The chlorine atoms on the benzene ring can be substituted with other functional groups under appropriate conditions.
Oxidation and Reduction Reactions: The compound can be oxidized or reduced to form different derivatives.
Common Reagents and Conditions:
Substitution Reactions: Reagents such as sodium hydroxide or potassium carbonate in the presence of a suitable solvent.
Oxidation Reactions: Oxidizing agents like potassium permanganate or hydrogen peroxide.
Reduction Reactions: Reducing agents such as sodium borohydride or lithium aluminum hydride.
Major Products: The major products formed from these reactions depend on the specific reagents and conditions used. For example, substitution reactions can yield various substituted benzamil derivatives, while oxidation and reduction reactions can produce different oxidized or reduced forms of the compound .
Scientific Research Applications
3’,4’-Dichlorobenzamil has a wide range of scientific research applications, including:
Chemistry: Used as a reagent in organic synthesis to study reaction mechanisms and develop new synthetic methodologies.
Biology: Employed in cellular and molecular biology research to investigate ion transport and signaling pathways.
Medicine: Studied for its potential therapeutic effects in conditions related to ion transport dysregulation, such as cardiac arrhythmias and hypertension.
Industry: Utilized in the development of new materials and chemical processes that require precise control of ion transport
Comparison with Similar Compounds
Amiloride: Another sodium-calcium exchanger inhibitor with similar ion transport modulation effects.
Benzamil: A derivative of amiloride with enhanced potency and specificity for sodium-calcium exchangers.
Phenamil: Another amiloride analog with similar inhibitory effects on ion transport.
Uniqueness of 3’,4’-Dichlorobenzamil: 3’,4’-Dichlorobenzamil is unique due to its specific substitution pattern on the benzene ring, which enhances its inhibitory effects on sodium-calcium exchangers compared to other similar compounds. This unique structure allows for more precise modulation of ion transport, making it a valuable tool in scientific research .
Properties
IUPAC Name |
3,5-diamino-6-chloro-N-[N'-[(3,4-dichlorophenyl)methyl]carbamimidoyl]pyrazine-2-carboxamide | |
---|---|---|
Source | PubChem | |
URL | https://pubchem.ncbi.nlm.nih.gov | |
Description | Data deposited in or computed by PubChem | |
InChI |
InChI=1S/C13H12Cl3N7O/c14-6-2-1-5(3-7(6)15)4-20-13(19)23-12(24)8-10(17)22-11(18)9(16)21-8/h1-3H,4H2,(H4,17,18,22)(H3,19,20,23,24) | |
Source | PubChem | |
URL | https://pubchem.ncbi.nlm.nih.gov | |
Description | Data deposited in or computed by PubChem | |
InChI Key |
OSHKWEFWXCCNJR-UHFFFAOYSA-N | |
Source | PubChem | |
URL | https://pubchem.ncbi.nlm.nih.gov | |
Description | Data deposited in or computed by PubChem | |
Canonical SMILES |
C1=CC(=C(C=C1CN=C(N)NC(=O)C2=C(N=C(C(=N2)Cl)N)N)Cl)Cl | |
Source | PubChem | |
URL | https://pubchem.ncbi.nlm.nih.gov | |
Description | Data deposited in or computed by PubChem | |
Molecular Formula |
C13H12Cl3N7O | |
Source | PubChem | |
URL | https://pubchem.ncbi.nlm.nih.gov | |
Description | Data deposited in or computed by PubChem | |
DSSTOX Substance ID |
DTXSID30922105 | |
Record name | 3,5-Diamino-6-chloro-N-{N-[(3,4-dichlorophenyl)methyl]carbamimidoyl}pyrazine-2-carboximidic acid | |
Source | EPA DSSTox | |
URL | https://comptox.epa.gov/dashboard/DTXSID30922105 | |
Description | DSSTox provides a high quality public chemistry resource for supporting improved predictive toxicology. | |
Molecular Weight |
388.6 g/mol | |
Source | PubChem | |
URL | https://pubchem.ncbi.nlm.nih.gov | |
Description | Data deposited in or computed by PubChem | |
CAS No. |
1166-01-4 | |
Record name | 3',4'-Dichlorobenzamil | |
Source | ChemIDplus | |
URL | https://pubchem.ncbi.nlm.nih.gov/substance/?source=chemidplus&sourceid=0001166014 | |
Description | ChemIDplus is a free, web search system that provides access to the structure and nomenclature authority files used for the identification of chemical substances cited in National Library of Medicine (NLM) databases, including the TOXNET system. | |
Record name | 3,5-Diamino-6-chloro-N-{N-[(3,4-dichlorophenyl)methyl]carbamimidoyl}pyrazine-2-carboximidic acid | |
Source | EPA DSSTox | |
URL | https://comptox.epa.gov/dashboard/DTXSID30922105 | |
Description | DSSTox provides a high quality public chemistry resource for supporting improved predictive toxicology. | |
Retrosynthesis Analysis
AI-Powered Synthesis Planning: Our tool employs the Template_relevance Pistachio, Template_relevance Bkms_metabolic, Template_relevance Pistachio_ringbreaker, Template_relevance Reaxys, Template_relevance Reaxys_biocatalysis model, leveraging a vast database of chemical reactions to predict feasible synthetic routes.
One-Step Synthesis Focus: Specifically designed for one-step synthesis, it provides concise and direct routes for your target compounds, streamlining the synthesis process.
Accurate Predictions: Utilizing the extensive PISTACHIO, BKMS_METABOLIC, PISTACHIO_RINGBREAKER, REAXYS, REAXYS_BIOCATALYSIS database, our tool offers high-accuracy predictions, reflecting the latest in chemical research and data.
Strategy Settings
Precursor scoring | Relevance Heuristic |
---|---|
Min. plausibility | 0.01 |
Model | Template_relevance |
Template Set | Pistachio/Bkms_metabolic/Pistachio_ringbreaker/Reaxys/Reaxys_biocatalysis |
Top-N result to add to graph | 6 |
Feasible Synthetic Routes
Q1: What is the primary molecular target of 3',4'-Dichlorobenzamil (DCB)?
A1: this compound (DCB) primarily targets the Na+/Ca2+ exchanger (NCX) [, , , , , ]. This exchanger plays a crucial role in maintaining cellular calcium homeostasis by transporting sodium and calcium ions across the cell membrane.
Q2: How does DCB interact with the Na+/Ca2+ exchanger?
A2: DCB acts as a potent inhibitor of the Na+/Ca2+ exchanger [, , , , ]. While the exact mechanism of inhibition varies with the direction of ion transport (inward vs. outward), studies suggest that DCB competitively inhibits Ca2+ binding to the exchanger, thereby blocking its activity [].
Q3: What are the downstream effects of DCB inhibiting the Na+/Ca2+ exchanger?
A3: Inhibition of the Na+/Ca2+ exchanger by DCB leads to a variety of downstream effects, depending on the cell type and context. Some key effects include:
- Increased intracellular Ca2+ levels: Blocking the efflux of Ca2+ via NCX can lead to an accumulation of intracellular calcium [, , ]. This is crucial in cell signaling and can impact processes like muscle contraction, neurotransmission, and hormone secretion.
- Decreased intracellular Na+ levels: Inhibition of NCX can also affect sodium levels, particularly in conditions where the inward mode of the exchanger is significant []. This can influence cellular excitability and other sodium-dependent processes.
- Modulation of Ca2+-dependent signaling pathways: By altering intracellular Ca2+ levels, DCB can indirectly influence numerous Ca2+-dependent signaling pathways [, , , ]. This can have broader consequences on cellular functions like gene expression, cell growth, and apoptosis.
Q4: Can you provide specific examples of how DCB affects cellular processes by targeting the Na+/Ca2+ exchanger?
A4: Certainly. Here are some examples:
- Platelet activation: DCB inhibits platelet aggregation and integrin αIIbβ3 activation, crucial steps in blood clotting, by interfering with Na+/Ca2+ exchange-mediated inside-out signaling [, ].
- Neurite outgrowth: Studies in PC12 cells suggest that DCB inhibits neurite outgrowth, potentially by interfering with Ca2+-dependent signaling pathways downstream of the Rho-ROCK pathway [, ].
- Fluid transport: DCB inhibits prostaglandin E2-stimulated fluid transport in cultured porcine thyroid cells, implying a role for Na+/Ca2+ exchange in this process [].
- Nitric oxide synthesis: DCB blocks the facilitating effect of Na+/Ca2+ exchange on Ca2+-dependent activation of endothelial nitric oxide synthase (eNOS) [, ]. This suggests a complex interplay between NCX, Ca2+ signaling, and eNOS activity in endothelial cells.
Q5: What is the molecular formula and weight of this compound (DCB)?
A5: The molecular formula of this compound is C16H15Cl2N7O and its molecular weight is 392.25 g/mol.
Q6: How do structural modifications of amiloride, the parent compound of DCB, affect its activity on the Na+/Ca2+ exchanger?
A6: Studies have shown that hydrophobic substitutions at the terminal nitrogen of the guanidinium group in amiloride significantly enhance its inhibitory potency towards the Na+/Ca2+ exchanger []. DCB, with its two chlorine atoms at the 3' and 4' positions of the benzoyl ring, exemplifies this trend, being a much more potent inhibitor compared to amiloride itself.
Q7: What in vitro models have been used to study the effects of DCB?
A7: Researchers have employed various in vitro models to investigate the effects of DCB, including:
- Membrane vesicles: Isolated cardiac sarcolemmal membrane vesicles have been instrumental in characterizing DCB's inhibitory effects on the Na+/Ca2+ exchanger [, ].
- Cultured cells: Studies utilizing cultured cells, such as human umbilical vein endothelial cells (HUVECs), mouse aortic smooth muscle cells (MASMCs), and PC12 cells, have provided insights into DCB's effects on various cellular processes like BKCa channel activity, neurite outgrowth, and nitric oxide synthesis [, , , , , ].
- Isolated tissues: Experiments on isolated tissues, like rat resistance arteries, rabbit kidney tubules, and porcine thyroid cells, have helped elucidate the role of Na+/Ca2+ exchange in regulating vascular tone, fluid transport, and hormone-stimulated responses [, , ].
Q8: What in vivo models have been used to study the effects of DCB?
A8: While the provided papers primarily focus on in vitro studies, a few mention in vivo experiments, for example:
- Rat model of hypertension: DCB was shown to reduce blood pressure in spontaneously hypertensive rats, suggesting a potential role in regulating blood pressure [].
- Guinea pig papillary muscle: In isolated, electrically stimulated guinea pig papillary muscles, DCB was found to decrease contractile force, providing evidence for its effects on cardiac muscle contractility [].
Q9: What are the potential cytotoxic effects of DCB?
A9: Studies have shown that DCB can induce cell death in isolated rat cardiac myocytes in a dose-dependent manner []. The specific mechanisms underlying this toxicity are not fully elucidated but likely involve disruption of cellular calcium homeostasis and subsequent activation of cell death pathways.
Q10: Are there alternative compounds that target the Na+/Ca2+ exchanger with potentially different selectivity profiles compared to DCB?
A10: Yes, several compounds target the Na+/Ca2+ exchanger with varying selectivity profiles. Some examples include:
- KB-R7943: This compound exhibits a different structure-activity relationship compared to amiloride derivatives and has shown promising results in inhibiting the Na+/Ca2+ exchanger in various cell types [, , ].
- Bepridil: Initially developed as a calcium channel blocker, bepridil has also been found to inhibit the Na+/Ca2+ exchanger [, , , ].
Disclaimer and Information on In-Vitro Research Products
Please be aware that all articles and product information presented on BenchChem are intended solely for informational purposes. The products available for purchase on BenchChem are specifically designed for in-vitro studies, which are conducted outside of living organisms. In-vitro studies, derived from the Latin term "in glass," involve experiments performed in controlled laboratory settings using cells or tissues. It is important to note that these products are not categorized as medicines or drugs, and they have not received approval from the FDA for the prevention, treatment, or cure of any medical condition, ailment, or disease. We must emphasize that any form of bodily introduction of these products into humans or animals is strictly prohibited by law. It is essential to adhere to these guidelines to ensure compliance with legal and ethical standards in research and experimentation.