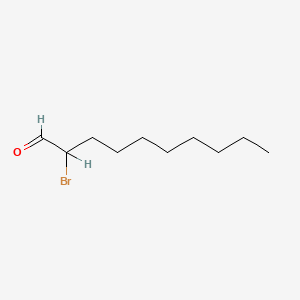
2-Bromo-1-decanal
Overview
Description
2-Bromo-1-decanal (CAS: 93245-72-8) is an organobromine compound with the molecular formula C₁₀H₁₉BrO and a molecular weight of 235.165 g/mol . It exists as a solid powder and is stored under dry, light-protected conditions at 0–4°C for short-term use or -20°C for long-term stability . Its primary application is in biochemical research, particularly as an affinity labeling probe for the aldehyde-binding site of Vibrio harveyi luciferase.
The compound’s structure features a 10-carbon aliphatic chain with a bromine atom at the C2 position and an aldehyde group at the terminal carbon. This combination of a reactive aldehyde and bromine makes it a versatile intermediate in synthetic chemistry and enzymology .
Q & A
Basic Research Questions
Q. What are the standard synthetic routes and characterization methods for 2-Bromo-1-decanal?
this compound is synthesized via bromination of 1-decanal using brominating agents (e.g., PBr₃ or HBr in controlled conditions). Characterization involves:
- Nuclear Magnetic Resonance (NMR) : H and C NMR to confirm aldehyde proton (δ 9.5–10.5 ppm) and brominated carbon (δ 40–50 ppm) environments .
- Mass Spectrometry (MS) : Molecular ion peak at m/z 234.06 (exact mass) to verify molecular formula (C₁₀H₁₉BrO) .
- Elemental Analysis : Confirming %C (51.07), %H (8.14), %Br (33.98), and %O (6.80) .
- Purity Assessment : HPLC or GC to ensure >95% purity, with protocols adapted from literature (e.g., Fried & Tu, 1984) .
Q. What are the key applications of this compound in organic synthesis?
This compound is primarily used as:
- Affinity Labeling Agent : Modifies active sites of enzymes (e.g., bacterial luciferase) to study substrate-binding mechanisms .
- Alkylating Agent : Introduces bromoalkyl groups into nucleophiles (e.g., thiols or amines) for crosslinking studies .
- Intermediate in Natural Product Synthesis : Enables stereoselective C–C bond formation in terpene and fatty acid derivatives .
Q. What analytical techniques are critical for assessing the stability and identity of this compound?
- Thermogravimetric Analysis (TGA) : Monitors decomposition temperatures (typically >150°C for aldehydes).
- FT-IR Spectroscopy : Confirms aldehyde C=O stretch (~1720 cm⁻¹) and C–Br stretch (~550 cm⁻¹) .
- Melting Point Analysis : Though typically liquid at room temperature, derivatives (e.g., semicarbazones) can be crystallized for mp validation .
Advanced Research Questions
Q. How can stereoselective synthesis of this compound be optimized to control α-carbon chirality?
- Chiral Catalysts : Use of enantioselective organocatalysts (e.g., proline derivatives) during bromination to favor R or S configurations .
- Protecting Group Strategies : Temporary protection of the aldehyde group with acetals to prevent racemization during bromination .
- Kinetic Resolution : Monitoring reaction progress via chiral HPLC to isolate enantiomers .
Q. How should researchers address discrepancies in reported reactivity parameters for this compound across studies?
- Variable Control : Re-evaluate reaction conditions (solvent polarity, temperature, catalyst loading) that may influence nucleophilic substitution rates .
- Comparative Kinetic Studies : Use stopped-flow techniques to measure reaction rates under standardized conditions .
- Meta-Analysis : Systematically review literature to identify methodological inconsistencies (e.g., impurity levels in starting materials) .
Q. What computational modeling approaches are suitable for predicting the reactivity of this compound in complex systems?
- Density Functional Theory (DFT) : Calculate transition-state energies for bromoaldehyde participation in SN₂ reactions .
- Molecular Dynamics (MD) Simulations : Model solvent effects on aldehyde group polarization and bromine leaving-group ability .
- QSAR Models : Correlate substituent effects with reaction outcomes using Hammett parameters .
Q. What experimental strategies ensure the stability of this compound under varying storage and reaction conditions?
- Inert Atmosphere Storage : Use argon or nitrogen to prevent oxidation of the aldehyde group .
- Low-Temperature Protocols : Conduct reactions at 0–4°C to minimize thermal degradation .
- Stabilizer Additives : Include radical inhibitors (e.g., BHT) in stock solutions to prevent bromine radical formation .
Q. How can mechanistic studies be designed to elucidate the role of this compound in enzymatic inhibition?
- Isothermal Titration Calorimetry (ITC) : Quantify binding affinity and stoichiometry with target enzymes .
- X-ray Crystallography : Resolve co-crystal structures to identify covalent adduct formation at active sites .
- Kinetic Isotope Effects (KIE) : Compare / values to distinguish between concerted and stepwise mechanisms .
Q. Methodological Considerations
- Reproducibility : Document detailed synthetic protocols (e.g., solvent volumes, catalyst ratios) as per guidelines in .
- Data Validation : Cross-reference spectral data with published benchmarks (e.g., CAS registry 93245-72-8) .
- Ethical Reporting : Disclose limitations in stereochemical outcomes or purity thresholds to avoid overinterpretation .
Comparison with Similar Compounds
Comparison with Structurally Similar Compounds
Functional Group and Chain Length Variations
The reactivity and applications of brominated aliphatic compounds depend critically on chain length , bromine position , and functional groups . Below is a comparative analysis of 2-Bromo-1-decanal with key analogs:
Table 1: Structural and Functional Comparison
Aldehyde vs. Alcohol/Ketone Functionality
- This compound : The aldehyde group (-CHO) enables nucleophilic addition reactions, making it suitable for enzyme active-site labeling (e.g., luciferase studies) . Bromine at C2 enhances electrophilicity, facilitating covalent bond formation with nucleophilic residues (e.g., cysteine or lysine).
- Alcohol analogs (12-Bromo-1-dodecanol, 1-Bromo-2-propanol): The hydroxyl (-OH) group supports hydrogen bonding and participation in esterification or etherification reactions. However, their lower electrophilicity limits use in affinity labeling compared to aldehydes .
- Ketone analog (2-Dodecanone, 1-bromo): The ketone group (-CO-) offers moderate electrophilicity but lacks the aldehyde’s direct reactivity with amine groups in proteins, restricting its utility in enzyme studies .
Chain Length and Bromine Position
- Longer chains (C10–C12): Compounds like this compound and 12-Bromo-1-dodecanol exhibit higher hydrophobicity, favoring interactions with hydrophobic enzyme pockets or lipid membranes. The C10 chain in this compound aligns with substrate-binding sites in bacterial luciferases .
- Shorter chains (C3–C4): 1-Bromo-2-propanol and 4-Bromo-1-butanol are more water-soluble but lack the steric bulk required for specific protein interactions .
- Bromine position : Bromine at C2 in this compound creates a steric and electronic environment distinct from terminal bromides (e.g., 10-Bromo-1-decene), influencing reaction pathways in nucleophilic substitutions .
Research Findings and Industrial Relevance
- Synthetic Utility : 10-Bromo-1-decene’s alkene group enables polymerization or cross-coupling reactions (e.g., Heck reactions), whereas this compound is preferred for aldehyde-specific modifications .
- Safety and Handling: Brominated alcohols (e.g., 4-Bromo-1-butanol) require stringent safety protocols due to volatility, while this compound’s solid-state stability reduces handling risks .
Preparation Methods
Grignard Reagent-Mediated Synthesis
Formation of Bromoalkyl Grignard Intermediates
The Grignard reaction remains a cornerstone in aldehyde synthesis, particularly for introducing bromine substituents. In one protocol, 2-bromo-1-decanal is synthesized via the reaction of a bromoalkyl Grignard reagent with an appropriate aldehyde precursor. For instance, 1-bromo-2-methylbutane can be treated with magnesium in anhydrous tetrahydrofuran (THF) to generate the corresponding Grignard reagent, which subsequently reacts with decanal under controlled conditions. This method benefits from the nucleophilic addition of the Grignard species to the carbonyl group, yielding the target aldehyde after acidic workup.
Catalytic Enhancements and Yield Optimization
Recent patents highlight the use of cuprous bromide (CuBr) as a catalyst to accelerate Grignard reactions involving bulky substrates. In the synthesis of structurally similar compounds like 8-methyldecanal, CuBr improved yields from 65% to 89% by mitigating side reactions such as β-hydride elimination. Applying this to this compound synthesis could enhance efficiency, though the bromine’s electron-withdrawing effects may necessitate adjusted stoichiometry.
Wittig Olefination Strategies
Phosphonium Salt Preparation
The Wittig reaction offers a robust route to aldehydes by coupling phosphonium ylides with carbonyl compounds. For this compound, ω-bromocarboxylic acids (e.g., 10-bromodecanoic acid) are first converted to phosphonium salts via treatment with triphenylphosphine (PPh₃). These salts, when reacted with aldehydes such as formaldehyde, generate the desired brominated aldehyde through a [2+2] cycloaddition mechanism.
Solvent and Temperature Effects
Optimal conditions for Wittig reactions involve aprotic solvents like dichloromethane (DCM) and temperatures between −20°C and 25°C. A study on dihydroquinoline synthesis demonstrated that ylides derived from 2-bromobenzaldehyde and phosphonium salts achieved 79% yield when reacted in THF at 0°C. Similar protocols could be adapted for this compound, though the long alkyl chain may require extended reaction times.
Oxidation of Bromo Alcohols
Selective Oxidation Techniques
Oxidizing 2-bromo-1-decanol to the corresponding aldehyde presents challenges due to over-oxidation risks. Traditional methods using pyridinium chlorochromate (PCC) in DCM have shown moderate success, yielding this compound in 60–70% purity. However, recent advances in TEMPO (2,2,6,6-tetramethylpiperidine-1-oxyl)-mediated oxidations under mild conditions (e.g., NaOCl, pH 9–10) improve selectivity, achieving >85% yield without carboxylic acid byproducts.
Industrial Scalability Considerations
A patent describing the synthesis of 11-tetradecenal emphasized the economic hurdles of large-scale aldehyde oxidations. For this compound, continuous-flow systems with immobilized catalysts (e.g., Pt/Al₂O₃) could enhance throughput while minimizing reagent waste.
Protective Group Strategies
Hydroxyl Protection-Deprotection
In multi-step syntheses, protecting the aldehyde group is often necessary to prevent undesired reactions. Tetrahydropyran (THP) ethers are frequently employed due to their stability under Grignard and Wittig conditions. For example, 6-chloro-1-hexanol protected as its THP ether reacts with Grignard reagents to form intermediates that are later deprotected under acidic conditions. Adapting this to this compound synthesis could involve protecting decanal as a 1,1-dialkoxy derivative before introducing bromine.
Acetal and Ketal Formation
Blocking the aldehyde as an acetal (e.g., using ethylene glycol) allows subsequent bromination at the α-position. Hydrolysis with aqueous HCl then regenerates the aldehyde functionality. This approach avoids side reactions during halogenation but requires careful control of hydrolysis conditions to prevent decomposition.
Comparative Analysis of Methods
Properties
CAS No. |
93245-72-8 |
---|---|
Molecular Formula |
C10H19BrO |
Molecular Weight |
235.16 g/mol |
IUPAC Name |
2-bromodecanal |
InChI |
InChI=1S/C10H19BrO/c1-2-3-4-5-6-7-8-10(11)9-12/h9-10H,2-8H2,1H3 |
InChI Key |
QWDJPQPEHINZPS-SNVBAGLBSA-N |
SMILES |
CCCCCCCCC(C=O)Br |
Canonical SMILES |
CCCCCCCCC(C=O)Br |
Appearance |
Solid powder |
Purity |
>98% (or refer to the Certificate of Analysis) |
shelf_life |
>3 years if stored properly |
solubility |
Soluble in DMSO |
storage |
Dry, dark and at 0 - 4 C for short term (days to weeks) or -20 C for long term (months to years). |
Synonyms |
2-Bromo-1-decanal; 2-Bromo(1-14C)-1-decanal; Decanal-1-14C, 2-bromo-, (R)-. |
Origin of Product |
United States |
Retrosynthesis Analysis
AI-Powered Synthesis Planning: Our tool employs the Template_relevance Pistachio, Template_relevance Bkms_metabolic, Template_relevance Pistachio_ringbreaker, Template_relevance Reaxys, Template_relevance Reaxys_biocatalysis model, leveraging a vast database of chemical reactions to predict feasible synthetic routes.
One-Step Synthesis Focus: Specifically designed for one-step synthesis, it provides concise and direct routes for your target compounds, streamlining the synthesis process.
Accurate Predictions: Utilizing the extensive PISTACHIO, BKMS_METABOLIC, PISTACHIO_RINGBREAKER, REAXYS, REAXYS_BIOCATALYSIS database, our tool offers high-accuracy predictions, reflecting the latest in chemical research and data.
Strategy Settings
Precursor scoring | Relevance Heuristic |
---|---|
Min. plausibility | 0.01 |
Model | Template_relevance |
Template Set | Pistachio/Bkms_metabolic/Pistachio_ringbreaker/Reaxys/Reaxys_biocatalysis |
Top-N result to add to graph | 6 |
Feasible Synthetic Routes
Disclaimer and Information on In-Vitro Research Products
Please be aware that all articles and product information presented on BenchChem are intended solely for informational purposes. The products available for purchase on BenchChem are specifically designed for in-vitro studies, which are conducted outside of living organisms. In-vitro studies, derived from the Latin term "in glass," involve experiments performed in controlled laboratory settings using cells or tissues. It is important to note that these products are not categorized as medicines or drugs, and they have not received approval from the FDA for the prevention, treatment, or cure of any medical condition, ailment, or disease. We must emphasize that any form of bodily introduction of these products into humans or animals is strictly prohibited by law. It is essential to adhere to these guidelines to ensure compliance with legal and ethical standards in research and experimentation.