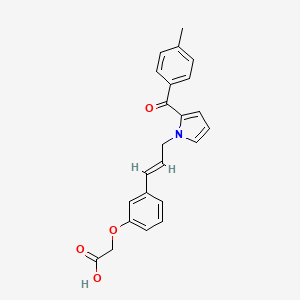
Pyrrole-derivative1
Overview
Description
Pyrrole-derivative1 is a heterocyclic organic compound that features a five-membered ring structure containing one nitrogen atom. Pyrrole and its derivatives are known for their significant biological and pharmacological activities. These compounds are widely used in medicinal chemistry due to their diverse therapeutic properties, including antibacterial, antifungal, anticancer, and anti-inflammatory activities .
Preparation Methods
Synthetic Routes and Reaction Conditions: Pyrrole-derivative1 can be synthesized through various synthetic routes. One common method involves the reaction of benzoin with antipyrine amine and malononitrile in a non-polar solvent . Another approach is the three-component synthesis, which involves the reaction of an aldehyde, an amine, and a β-dicarbonyl compound under mild reaction conditions . The reactions catalyzed by proline, copper oxides, and oxones have been shown to be effective for synthesizing pyrrole derivatives with excellent yields .
Industrial Production Methods: In industrial settings, the production of this compound often involves multicomponent reactions (MCRs) due to their efficiency and simplicity. These reactions allow for the rapid assembly of complex molecules from simple starting materials. The use of green chemistry principles, such as solvent-free methods and microwave-aided synthesis, is also gaining popularity in the industrial production of pyrrole derivatives .
Chemical Reactions Analysis
Types of Reactions: Pyrrole-derivative1 undergoes various chemical reactions, including oxidation, reduction, and substitution reactions. For example, the oxidation of pyrrole derivatives can be achieved using reagents such as potassium permanganate or hydrogen peroxide . Reduction reactions often involve the use of reducing agents like lithium aluminum hydride or sodium borohydride .
Common Reagents and Conditions: Common reagents used in the reactions of this compound include acids, bases, and transition metal catalysts. For instance, the N-alkylation of pyrroles can be catalyzed by iridium complexes, leading to the formation of tetrahydropyrrolo[1,2-c]pyrimidine derivatives . The reaction conditions typically involve mild temperatures and atmospheric pressure to ensure high yields and selectivity .
Major Products Formed: The major products formed from the reactions of this compound depend on the specific reaction conditions and reagents used. For example, the oxidation of pyrrole derivatives can lead to the formation of pyrrole-2,5-dicarboxylic acid, while reduction reactions can yield pyrrolidine derivatives .
Scientific Research Applications
Pyrrole-derivative1 has a wide range of scientific research applications. In chemistry, it is used as a building block for the synthesis of more complex molecules . In biology, pyrrole derivatives are studied for their potential as enzyme inhibitors and antimicrobial agents . In medicine, these compounds are investigated for their anticancer, anti-inflammatory, and neuroprotective properties . Additionally, pyrrole derivatives find applications in the industry as components of organic dyes, conjugated polymers, and optoelectronic devices .
Mechanism of Action
The mechanism of action of pyrrole-derivative1 involves its interaction with specific molecular targets and pathways. For instance, some pyrrole derivatives act as kinase inhibitors by binding to the active site of the enzyme and preventing its activity . This inhibition can lead to the suppression of cell proliferation and the induction of apoptosis in cancer cells . The exact molecular targets and pathways involved depend on the specific structure and functional groups of the pyrrole derivative .
Comparison with Similar Compounds
Pyrrole-derivative1 can be compared with other similar compounds, such as pyrrolo[3,2-b]quinoxaline derivatives and pyrrolo[3,2-b]pyrroles . These compounds share a similar core structure but differ in their functional groups and biological activities. For example, pyrrolo[3,2-b]quinoxaline derivatives are known for their kinase inhibitory activity, while pyrrolo[3,2-b]pyrroles exhibit strong fluorescent properties . The uniqueness of this compound lies in its specific combination of functional groups, which confer distinct biological and pharmacological properties .
Properties
IUPAC Name |
2-[3-[(E)-3-[2-(4-methylbenzoyl)pyrrol-1-yl]prop-1-enyl]phenoxy]acetic acid | |
---|---|---|
Source | PubChem | |
URL | https://pubchem.ncbi.nlm.nih.gov | |
Description | Data deposited in or computed by PubChem | |
InChI |
InChI=1S/C23H21NO4/c1-17-9-11-19(12-10-17)23(27)21-8-4-14-24(21)13-3-6-18-5-2-7-20(15-18)28-16-22(25)26/h2-12,14-15H,13,16H2,1H3,(H,25,26)/b6-3+ | |
Source | PubChem | |
URL | https://pubchem.ncbi.nlm.nih.gov | |
Description | Data deposited in or computed by PubChem | |
InChI Key |
CNBLGGYQBCKUKJ-ZZXKWVIFSA-N | |
Source | PubChem | |
URL | https://pubchem.ncbi.nlm.nih.gov | |
Description | Data deposited in or computed by PubChem | |
Canonical SMILES |
CC1=CC=C(C=C1)C(=O)C2=CC=CN2CC=CC3=CC(=CC=C3)OCC(=O)O | |
Source | PubChem | |
URL | https://pubchem.ncbi.nlm.nih.gov | |
Description | Data deposited in or computed by PubChem | |
Isomeric SMILES |
CC1=CC=C(C=C1)C(=O)C2=CC=CN2C/C=C/C3=CC(=CC=C3)OCC(=O)O | |
Source | PubChem | |
URL | https://pubchem.ncbi.nlm.nih.gov | |
Description | Data deposited in or computed by PubChem | |
Molecular Formula |
C23H21NO4 | |
Source | PubChem | |
URL | https://pubchem.ncbi.nlm.nih.gov | |
Description | Data deposited in or computed by PubChem | |
Molecular Weight |
375.4 g/mol | |
Source | PubChem | |
URL | https://pubchem.ncbi.nlm.nih.gov | |
Description | Data deposited in or computed by PubChem | |
Retrosynthesis Analysis
AI-Powered Synthesis Planning: Our tool employs the Template_relevance Pistachio, Template_relevance Bkms_metabolic, Template_relevance Pistachio_ringbreaker, Template_relevance Reaxys, Template_relevance Reaxys_biocatalysis model, leveraging a vast database of chemical reactions to predict feasible synthetic routes.
One-Step Synthesis Focus: Specifically designed for one-step synthesis, it provides concise and direct routes for your target compounds, streamlining the synthesis process.
Accurate Predictions: Utilizing the extensive PISTACHIO, BKMS_METABOLIC, PISTACHIO_RINGBREAKER, REAXYS, REAXYS_BIOCATALYSIS database, our tool offers high-accuracy predictions, reflecting the latest in chemical research and data.
Strategy Settings
Precursor scoring | Relevance Heuristic |
---|---|
Min. plausibility | 0.01 |
Model | Template_relevance |
Template Set | Pistachio/Bkms_metabolic/Pistachio_ringbreaker/Reaxys/Reaxys_biocatalysis |
Top-N result to add to graph | 6 |
Feasible Synthetic Routes
Disclaimer and Information on In-Vitro Research Products
Please be aware that all articles and product information presented on BenchChem are intended solely for informational purposes. The products available for purchase on BenchChem are specifically designed for in-vitro studies, which are conducted outside of living organisms. In-vitro studies, derived from the Latin term "in glass," involve experiments performed in controlled laboratory settings using cells or tissues. It is important to note that these products are not categorized as medicines or drugs, and they have not received approval from the FDA for the prevention, treatment, or cure of any medical condition, ailment, or disease. We must emphasize that any form of bodily introduction of these products into humans or animals is strictly prohibited by law. It is essential to adhere to these guidelines to ensure compliance with legal and ethical standards in research and experimentation.