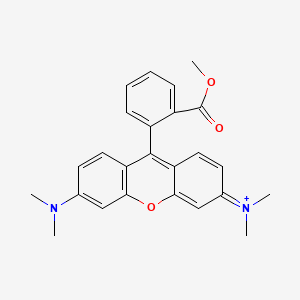
TMRM
Overview
Description
Tetramethylrhodamine, methyl ester, perchlorate (TMRM) is a cell-permeant, cationic, red-orange fluorescent dye that is readily sequestered by active mitochondria. It is widely used as a mitochondrial membrane potential indicator in various biological and medical research applications. This compound accumulates in active mitochondria with intact membrane potentials, emitting a bright signal if the cells are healthy and have functioning mitochondria. Upon loss of the mitochondrial membrane potential, this compound accumulation ceases, and the signal dims or disappears .
Preparation Methods
Synthetic Routes and Reaction Conditions: TMRM is synthesized through a series of chemical reactions involving the introduction of methyl ester groups to the tetramethylrhodamine structure. The synthesis typically involves the esterification of tetramethylrhodamine with methanol in the presence of an acid catalyst. The reaction conditions include maintaining a controlled temperature and pH to ensure the successful formation of the methyl ester derivative.
Industrial Production Methods: In industrial settings, this compound is produced in larger quantities using optimized reaction conditions to ensure high yield and purity. The process involves the use of automated reactors and precise control of reaction parameters such as temperature, pressure, and pH. The final product is purified through techniques such as crystallization and chromatography to obtain the desired quality .
Types of Reactions:
Oxidation: this compound can undergo oxidation reactions, leading to the formation of oxidized derivatives.
Reduction: The compound can also participate in reduction reactions, resulting in reduced forms of this compound.
Substitution: this compound can undergo substitution reactions where functional groups on the molecule are replaced with other groups.
Common Reagents and Conditions:
Oxidation: Common oxidizing agents such as hydrogen peroxide or potassium permanganate can be used under controlled conditions.
Reduction: Reducing agents like sodium borohydride or lithium aluminum hydride are typically employed.
Substitution: Various nucleophiles or electrophiles can be used depending on the desired substitution reaction.
Major Products Formed: The major products formed from these reactions include various oxidized, reduced, and substituted derivatives of this compound, each with distinct chemical and physical properties .
Scientific Research Applications
TMRM has a wide range of applications in scientific research, including but not limited to:
Chemistry: Used as a fluorescent probe to study chemical reactions and molecular interactions.
Biology: Employed to assess mitochondrial membrane potential in live cells, providing insights into cellular health and function.
Medicine: Utilized in research on mitochondrial diseases, apoptosis, and other cellular processes.
Industry: Applied in high-throughput screening and high-content analysis for drug discovery and development
Mechanism of Action
TMRM exerts its effects by accumulating in active mitochondria with intact membrane potentials. The accumulation is driven by the electrochemical gradient across the mitochondrial membrane. Healthy mitochondria maintain a difference in electrical potential between the interior and exterior of the organelle, referred to as the membrane potential. This compound, being a cationic dye, is attracted to the negatively charged interior of the mitochondria. Upon loss of the mitochondrial membrane potential, this compound accumulation ceases, and the signal dims or disappears .
Comparison with Similar Compounds
Tetramethylrhodamine, Ethyl Ester (TMRE): Similar to TMRM but with ethyl ester groups instead of methyl ester groups.
Rhodamine 123: Another mitochondrial dye with different spectral properties.
MitoTracker Red: A commercially available mitochondrial dye with distinct chemical properties.
Comparison: this compound is unique in its ability to provide a bright and stable signal in live cell imaging, making it highly suitable for dynamic studies of mitochondrial membrane potential. Compared to TMRE, this compound has slightly different spectral properties, which can be advantageous in certain experimental setups. Rhodamine 123 and MitoTracker Red also serve similar purposes but may differ in terms of their accumulation kinetics, photostability, and compatibility with other fluorescent dyes .
Biological Activity
Tetramethylrhodamine methyl ester (TMRM) is a fluorescent dye widely used in biological research, particularly for assessing mitochondrial membrane potential (). This compound has gained significant attention due to its ability to selectively accumulate in mitochondria, making it an invaluable tool for studying mitochondrial function and dynamics.
This compound operates primarily through two modes: quenching and non-quenching .
- Quenching Mode : In this mode, cells are loaded with a high concentration of this compound, which accumulates in the mitochondrial matrix. The fluorescence of this compound is quenched as it aggregates within the matrix. When mitochondrial depolarization occurs, this compound leaks out, leading to an increase in cytoplasmic fluorescence.
- Non-Quenching Mode : At lower concentrations (5–20 nM), this compound accumulates in the mitochondria without quenching. Here, mitochondrial depolarization results in a decrease in fluorescence signal, allowing for sensitive measurements of changes in .
Applications in Research
This compound is utilized extensively in various research settings:
- Mitochondrial Function Studies : It is commonly used to measure kinetics in live cells using fluorescence microscopy. Recent advancements include its application in two-photon microscopy and super-resolution microscopy, enabling detailed studies of mitochondrial heterogeneity .
- Toxicology Assessments : this compound has been employed to assess mitochondrial toxicity induced by various agents, including high glucose levels which can lead to mitochondrial calcium overload and subsequent cell death .
- Cellular Dynamics : Studies have demonstrated that this compound can reveal dynamic changes in mitochondrial morphology and function under different physiological and pathological conditions .
Comparative Studies
Recent comparative studies have highlighted the effectiveness of this compound relative to other fluorescent probes:
Fluorescent Probe | Sensitivity to | Application |
---|---|---|
This compound | High | Mitochondrial potential measurement |
Mitotracker Red CMXros | Moderate | Mitochondrial morphology analysis |
Mitotracker Green FM | Low | General mitochondrial studies |
These findings suggest that this compound is superior for integrated analysis of both mitochondrial potential and morphology compared to other probes .
Study 1: Mitochondrial Dysfunction in Diabetes
In a study examining the effects of high glucose on mitochondrial function, researchers utilized this compound to measure changes in . The results indicated significant depolarization associated with increased reactive oxygen species (ROS) production, highlighting the role of this compound as a critical tool for understanding metabolic diseases .
Study 2: Cancer Cell Metabolism
Another study focused on the role of this compound in assessing mitochondrial function in cancer cells. By measuring , researchers were able to demonstrate how certain therapeutic agents induce mitochondrial depolarization, leading to apoptosis. This underscores the utility of this compound in cancer research, particularly for evaluating drug efficacy .
Properties
IUPAC Name |
[6-(dimethylamino)-9-(2-methoxycarbonylphenyl)xanthen-3-ylidene]-dimethylazanium | |
---|---|---|
Source | PubChem | |
URL | https://pubchem.ncbi.nlm.nih.gov | |
Description | Data deposited in or computed by PubChem | |
InChI |
InChI=1S/C25H25N2O3/c1-26(2)16-10-12-20-22(14-16)30-23-15-17(27(3)4)11-13-21(23)24(20)18-8-6-7-9-19(18)25(28)29-5/h6-15H,1-5H3/q+1 | |
Source | PubChem | |
URL | https://pubchem.ncbi.nlm.nih.gov | |
Description | Data deposited in or computed by PubChem | |
InChI Key |
WAWRKBQQBUDAMY-UHFFFAOYSA-N | |
Source | PubChem | |
URL | https://pubchem.ncbi.nlm.nih.gov | |
Description | Data deposited in or computed by PubChem | |
Canonical SMILES |
CN(C)C1=CC2=C(C=C1)C(=C3C=CC(=[N+](C)C)C=C3O2)C4=CC=CC=C4C(=O)OC | |
Source | PubChem | |
URL | https://pubchem.ncbi.nlm.nih.gov | |
Description | Data deposited in or computed by PubChem | |
Molecular Formula |
C25H25N2O3+ | |
Source | PubChem | |
URL | https://pubchem.ncbi.nlm.nih.gov | |
Description | Data deposited in or computed by PubChem | |
Molecular Weight |
401.5 g/mol | |
Source | PubChem | |
URL | https://pubchem.ncbi.nlm.nih.gov | |
Description | Data deposited in or computed by PubChem | |
Retrosynthesis Analysis
AI-Powered Synthesis Planning: Our tool employs the Template_relevance Pistachio, Template_relevance Bkms_metabolic, Template_relevance Pistachio_ringbreaker, Template_relevance Reaxys, Template_relevance Reaxys_biocatalysis model, leveraging a vast database of chemical reactions to predict feasible synthetic routes.
One-Step Synthesis Focus: Specifically designed for one-step synthesis, it provides concise and direct routes for your target compounds, streamlining the synthesis process.
Accurate Predictions: Utilizing the extensive PISTACHIO, BKMS_METABOLIC, PISTACHIO_RINGBREAKER, REAXYS, REAXYS_BIOCATALYSIS database, our tool offers high-accuracy predictions, reflecting the latest in chemical research and data.
Strategy Settings
Precursor scoring | Relevance Heuristic |
---|---|
Min. plausibility | 0.01 |
Model | Template_relevance |
Template Set | Pistachio/Bkms_metabolic/Pistachio_ringbreaker/Reaxys/Reaxys_biocatalysis |
Top-N result to add to graph | 6 |
Feasible Synthetic Routes
Disclaimer and Information on In-Vitro Research Products
Please be aware that all articles and product information presented on BenchChem are intended solely for informational purposes. The products available for purchase on BenchChem are specifically designed for in-vitro studies, which are conducted outside of living organisms. In-vitro studies, derived from the Latin term "in glass," involve experiments performed in controlled laboratory settings using cells or tissues. It is important to note that these products are not categorized as medicines or drugs, and they have not received approval from the FDA for the prevention, treatment, or cure of any medical condition, ailment, or disease. We must emphasize that any form of bodily introduction of these products into humans or animals is strictly prohibited by law. It is essential to adhere to these guidelines to ensure compliance with legal and ethical standards in research and experimentation.