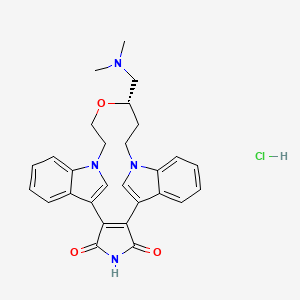
Ruboxistaurin Hydrochloride
Overview
Description
LY333531 (hydrochloride), also known as Ruboxistaurin hydrochloride, is a potent and selective inhibitor of protein kinase C beta (PKCβ). It is primarily used in scientific research to study the role of PKCβ in various biological processes and diseases. The compound has shown promise in the treatment of diabetic complications, such as diabetic retinopathy, diabetic peripheral neuropathy, and diabetic nephropathy .
Mechanism of Action
Target of Action
Ruboxistaurin Hydrochloride, also known as LY 333531 Hydrochloride, is primarily targeted towards Protein Kinase C beta (PKCβ) . PKCβ is a member of the protein kinase C family of serine/threonine kinases, which are involved in a multitude of cellular processes such as proliferation, differentiation, and secretion .
Biochemical Pathways
The inhibition of PKCβ by this compound affects various biochemical pathways. One such pathway involves the regulation of neuronal nitric oxide synthase (nNOS)-cGMP system, which may be involved in diabetic hyperalgesia .
Pharmacokinetics
The pharmacokinetics of this compound were found to be linear within the dose range and duration of the study . Steady state was achieved after 7–21 days with twice-daily dosing . Ruboxistaurin given in the evening had lower plasma concentrations and a longer time to maximal concentration compared with the morning dosage . It’s important to note that co-administration with known CYP3A4 inducing agents may decrease the concentrations of Ruboxistaurin and N-desmethyl-ruboxistaurin .
Result of Action
The inhibition of PKCβ by this compound can lead to various molecular and cellular effects. For instance, it has been shown to ameliorate the decrease in the nNOS-cGMP system, which could potentially alleviate diabetic hyperalgesia .
Action Environment
The action, efficacy, and stability of this compound can be influenced by various environmental factors. For example, the time of administration can affect its plasma concentrations . Furthermore, the presence of CYP3A4 inducing agents can significantly alter its pharmacokinetics .
Biochemical Analysis
Biochemical Properties
Ruboxistaurin Hydrochloride competitively inhibits PKCβI and PKCβII . The IC50 values are 4.7 and 5.9 nM respectively . It exhibits selectivity for PKC over other ATP-dependent kinases, including protein kinase A, casein kinase, and src .
Cellular Effects
This compound has significant effects on various types of cells and cellular processes. PKCβ isoforms, which this compound inhibits, are overexpressed in states of oxidative stress, especially in association with diabetes . In diabetic rats, this compound improves blood flow and leukocyte entrapment in the retina, and protects against myocardial hypertrophy and cardiac dysfunction .
Molecular Mechanism
The molecular mechanism of this compound involves its competitive inhibition of PKCβI and PKCβII . It binds to these enzymes and prevents them from catalyzing their respective reactions, thereby influencing various cellular and molecular processes .
Temporal Effects in Laboratory Settings
It is known that it is soluble in DMSO to 20 mM , indicating its stability in this solvent
Metabolic Pathways
This compound is involved in the PKCβ signaling pathway . It interacts with PKCβI and PKCβII enzymes, influencing metabolic flux and metabolite levels .
Preparation Methods
Synthetic Routes and Reaction Conditions
The synthesis of LY333531 (hydrochloride) involves multiple steps, starting from commercially available starting materialsThe final step involves the formation of the hydrochloride salt to improve the compound’s solubility and stability .
Industrial Production Methods
Industrial production of LY333531 (hydrochloride) follows similar synthetic routes as the laboratory synthesis but is optimized for large-scale production. This includes the use of more efficient catalysts, optimized reaction conditions, and purification techniques to ensure high yield and purity of the final product .
Chemical Reactions Analysis
Types of Reactions
LY333531 (hydrochloride) undergoes various chemical reactions, including:
Oxidation: The compound can be oxidized under specific conditions to form oxidized derivatives.
Reduction: Reduction reactions can be used to modify the functional groups on the compound.
Substitution: Substitution reactions can be employed to introduce different substituents on the aromatic rings.
Common Reagents and Conditions
Oxidation: Common oxidizing agents such as hydrogen peroxide or potassium permanganate can be used.
Reduction: Reducing agents like sodium borohydride or lithium aluminum hydride are typically employed.
Substitution: Various electrophiles and nucleophiles can be used in substitution reactions, depending on the desired modification.
Major Products Formed
The major products formed from these reactions depend on the specific reagents and conditions used. For example, oxidation may yield oxidized derivatives with altered biological activity, while substitution reactions can produce analogs with different pharmacological properties .
Scientific Research Applications
LY333531 (hydrochloride) has a wide range of scientific research applications, including:
Chemistry: Used as a tool compound to study the role of PKCβ in various chemical reactions and pathways.
Biology: Employed in cell biology research to investigate the effects of PKCβ inhibition on cellular processes such as proliferation, differentiation, and apoptosis.
Medicine: Investigated for its potential therapeutic effects in treating diabetic complications, cancer, and other diseases involving PKCβ dysregulation.
Industry: Utilized in the development of new drugs and therapeutic agents targeting PKCβ.
Comparison with Similar Compounds
Similar Compounds
Enzastaurin: Another PKCβ inhibitor with a broader spectrum of activity against other PKC isoforms.
Bisindolylmaleimide I: A non-selective PKC inhibitor that targets multiple PKC isoforms.
Go 6983: A pan-PKC inhibitor that inhibits several PKC isoforms with varying potency.
Uniqueness
LY333531 (hydrochloride) is unique in its high selectivity for PKCβ over other PKC isoforms. This selectivity makes it a valuable tool for studying the specific role of PKCβ in various biological processes and diseases. Additionally, its oral bioavailability and favorable pharmacokinetic properties make it suitable for in vivo studies .
Properties
IUPAC Name |
(18S)-18-[(dimethylamino)methyl]-17-oxa-4,14,21-triazahexacyclo[19.6.1.17,14.02,6.08,13.022,27]nonacosa-1(28),2(6),7(29),8,10,12,22,24,26-nonaene-3,5-dione;hydrochloride | |
---|---|---|
Source | PubChem | |
URL | https://pubchem.ncbi.nlm.nih.gov | |
Description | Data deposited in or computed by PubChem | |
InChI |
InChI=1S/C28H28N4O3.ClH/c1-30(2)15-18-11-12-31-16-21(19-7-3-5-9-23(19)31)25-26(28(34)29-27(25)33)22-17-32(13-14-35-18)24-10-6-4-8-20(22)24;/h3-10,16-18H,11-15H2,1-2H3,(H,29,33,34);1H/t18-;/m0./s1 | |
Source | PubChem | |
URL | https://pubchem.ncbi.nlm.nih.gov | |
Description | Data deposited in or computed by PubChem | |
InChI Key |
NYQIEYDJYFVLPO-FERBBOLQSA-N | |
Source | PubChem | |
URL | https://pubchem.ncbi.nlm.nih.gov | |
Description | Data deposited in or computed by PubChem | |
Canonical SMILES |
CN(C)CC1CCN2C=C(C3=CC=CC=C32)C4=C(C5=CN(CCO1)C6=CC=CC=C65)C(=O)NC4=O.Cl | |
Source | PubChem | |
URL | https://pubchem.ncbi.nlm.nih.gov | |
Description | Data deposited in or computed by PubChem | |
Isomeric SMILES |
CN(C)C[C@@H]1CCN2C=C(C3=CC=CC=C32)C4=C(C5=CN(CCO1)C6=CC=CC=C65)C(=O)NC4=O.Cl | |
Source | PubChem | |
URL | https://pubchem.ncbi.nlm.nih.gov | |
Description | Data deposited in or computed by PubChem | |
Molecular Formula |
C28H29ClN4O3 | |
Source | PubChem | |
URL | https://pubchem.ncbi.nlm.nih.gov | |
Description | Data deposited in or computed by PubChem | |
Molecular Weight |
505.0 g/mol | |
Source | PubChem | |
URL | https://pubchem.ncbi.nlm.nih.gov | |
Description | Data deposited in or computed by PubChem | |
CAS No. |
169939-93-9 | |
Record name | Ruboxistaurin hydrochloride | |
Source | ChemIDplus | |
URL | https://pubchem.ncbi.nlm.nih.gov/substance/?source=chemidplus&sourceid=0169939939 | |
Description | ChemIDplus is a free, web search system that provides access to the structure and nomenclature authority files used for the identification of chemical substances cited in National Library of Medicine (NLM) databases, including the TOXNET system. | |
Record name | RUBOXISTAURIN HYDROCHLORIDE | |
Source | FDA Global Substance Registration System (GSRS) | |
URL | https://gsrs.ncats.nih.gov/ginas/app/beta/substances/6496V4OCZN | |
Description | The FDA Global Substance Registration System (GSRS) enables the efficient and accurate exchange of information on what substances are in regulated products. Instead of relying on names, which vary across regulatory domains, countries, and regions, the GSRS knowledge base makes it possible for substances to be defined by standardized, scientific descriptions. | |
Explanation | Unless otherwise noted, the contents of the FDA website (www.fda.gov), both text and graphics, are not copyrighted. They are in the public domain and may be republished, reprinted and otherwise used freely by anyone without the need to obtain permission from FDA. Credit to the U.S. Food and Drug Administration as the source is appreciated but not required. | |
Retrosynthesis Analysis
AI-Powered Synthesis Planning: Our tool employs the Template_relevance Pistachio, Template_relevance Bkms_metabolic, Template_relevance Pistachio_ringbreaker, Template_relevance Reaxys, Template_relevance Reaxys_biocatalysis model, leveraging a vast database of chemical reactions to predict feasible synthetic routes.
One-Step Synthesis Focus: Specifically designed for one-step synthesis, it provides concise and direct routes for your target compounds, streamlining the synthesis process.
Accurate Predictions: Utilizing the extensive PISTACHIO, BKMS_METABOLIC, PISTACHIO_RINGBREAKER, REAXYS, REAXYS_BIOCATALYSIS database, our tool offers high-accuracy predictions, reflecting the latest in chemical research and data.
Strategy Settings
Precursor scoring | Relevance Heuristic |
---|---|
Min. plausibility | 0.01 |
Model | Template_relevance |
Template Set | Pistachio/Bkms_metabolic/Pistachio_ringbreaker/Reaxys/Reaxys_biocatalysis |
Top-N result to add to graph | 6 |
Feasible Synthetic Routes
Disclaimer and Information on In-Vitro Research Products
Please be aware that all articles and product information presented on BenchChem are intended solely for informational purposes. The products available for purchase on BenchChem are specifically designed for in-vitro studies, which are conducted outside of living organisms. In-vitro studies, derived from the Latin term "in glass," involve experiments performed in controlled laboratory settings using cells or tissues. It is important to note that these products are not categorized as medicines or drugs, and they have not received approval from the FDA for the prevention, treatment, or cure of any medical condition, ailment, or disease. We must emphasize that any form of bodily introduction of these products into humans or animals is strictly prohibited by law. It is essential to adhere to these guidelines to ensure compliance with legal and ethical standards in research and experimentation.