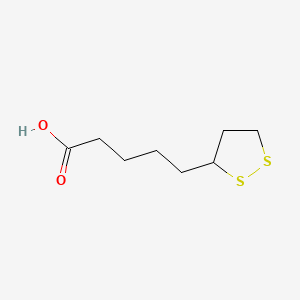
Thioctic acid
Overview
Description
Thioctic acid, also known as alpha-lipoic acid, is an organosulfur compound derived from octanoic acid. It is a naturally occurring compound found in plants, animals, and humans. This compound is known for its potent antioxidant properties and plays a crucial role in mitochondrial bioenergetic reactions. It is essential for aerobic metabolism and is available as a dietary supplement or pharmaceutical drug in some countries .
Mechanism of Action
Thioctic acid, also known as α-lipoic acid (ALA), is an organosulfur compound derived from caprylic acid (octanoic acid) . It has various properties, including antioxidant potential, and is widely used as a dietary supplement or pharmaceutical drug .
Target of Action
This compound primarily targets enzymes or classes of enzymes: pyruvate dehydrogenase, α-ketoglutarate dehydrogenase, the glycine cleavage system, branched-chain alpha-keto acid dehydrogenase, and the α-oxo (keto)adipate dehydrogenase . These enzymes play crucial roles in various biochemical pathways, including the citric acid cycle . HDAC1, HDAC2, HDAC3, HDAC6, HDAC8, and HDAC10 are also targets of the reduced form of this compound .
Mode of Action
This compound acts as an antioxidant, protecting cells from damage . It improves blood flow to peripheral nerves, easing painful symptoms, and increases nerve conduction velocity . It is generally involved in oxidative decarboxylations of keto acids and is presented as a growth factor for some organisms .
Biochemical Pathways
This compound is a cofactor for several enzymes, playing a critical role in the citric acid cycle . It is involved in the oxidative decarboxylation of keto acids . It also regulates glycine concentrations through the glycine cleavage system .
Pharmacokinetics
This compound is quickly absorbed from the gastrointestinal tract . . Data suggests that this compound has a short half-life and bioavailability (about 30%) triggered by its hepatic degradation, reduced solubility, and instability in the stomach . The use of various innovative formulations has greatly improved this compound’s bioavailability .
Result of Action
This compound demonstrates promise in alleviating symptoms of diabetic neuropathy, a common complication of diabetes . By combating lipid peroxidation and inhibiting aldose reductase, this compound offers a multi-faceted approach to diabetes management . It also has the potential to treat neurodegenerative disorders .
Action Environment
The action of this compound can be influenced by environmental factors. For instance, light-dependent decomposition of this compound can lead to the formation of H2S, a signal molecule that plays an important role in cellular regulatory processes . The manufacturing features of this compound tablets, such as the high content of the pharmaceutical substance in tablets (up to 600 mg), the need to use a minimum amount of excipients (from 27 to 51%), poor flowability, and low melting point of the pharmaceutical substance, should be taken into account .
Biochemical Analysis
Biochemical Properties
Thioctic acid interacts with various enzymes and proteins within the body. It serves as a cofactor for five key enzymes: pyruvate dehydrogenase, α-ketoglutarate dehydrogenase, the glycine cleavage system, branched-chain alpha-keto acid dehydrogenase, and the α-oxo (keto)adipate dehydrogenase . These enzymes are critical to the citric acid cycle and the regulation of glycine concentrations . This compound’s role in these biochemical reactions underscores its importance in energy metabolism.
Cellular Effects
This compound exerts significant effects on various types of cells and cellular processes. It is known to scavenge reactive oxygen species, thereby reducing oxidative stress within cells . This compound also influences cell function by impacting cell signaling pathways, gene expression, and cellular metabolism . Its antioxidant activity helps slow down cellular damage, a root cause of many diseases .
Molecular Mechanism
At the molecular level, this compound exerts its effects through several mechanisms. It acts as a chelating agent for metal ions, a quenching agent for reactive oxygen species, and a reducing agent for the oxidized form of glutathione and vitamins C and E . This compound’s antioxidant potential and free radical scavenging activity are largely attributed to the sulfhydryl (-SH) group of molecules, which has the greatest electron donating activity .
Temporal Effects in Laboratory Settings
In laboratory settings, the effects of this compound can change over time. For instance, subchronic administrations of this compound have been shown to cause overall body weight loss, motorial impairment, and mass loss in some organs
Dosage Effects in Animal Models
The effects of this compound can vary with different dosages in animal models. For example, a study on spontaneously hypertensive rats showed that the highest doses of all this compound compounds negatively affected motorial ability . The study also found that this compound’s LDmin (minimum lethal dose) was higher than other compounds .
Metabolic Pathways
This compound is involved in several metabolic pathways. It is a necessary cofactor for mitochondrial α-ketoacid dehydrogenases, which are essential for aerobic metabolism . This compound’s involvement in these metabolic pathways underscores its critical role in energy production and metabolism.
Subcellular Localization
Current research suggests that this compound is present in every cell inside the body , indicating a widespread subcellular distribution
Preparation Methods
Synthetic Routes and Reaction Conditions: Thioctic acid can be synthesized through various methods. One common method involves the reaction of octanoic acid with sulfur to form the disulfide bond. Another method includes the use of alcoholized sodium in an alcohol solution, followed by heating and stirring to form high-purity this compound .
Industrial Production Methods: In industrial settings, this compound is produced by dispersing the raw material in water, adding an alcohol solution of alcoholized sodium, and heating the mixture. The solution is then filtered, and the filtrate is treated with active carbon or macroporous adsorption resin. The final product is obtained by adjusting the pH, heating, and crystallization .
Chemical Reactions Analysis
Types of Reactions: Thioctic acid undergoes various chemical reactions, including oxidation, reduction, and substitution. It contains two sulfur atoms connected by a disulfide bond, which can be reduced to form dihydrolipoic acid .
Common Reagents and Conditions:
Oxidation: this compound can be oxidized to form its disulfide form.
Reduction: It can be reduced using reducing agents to form dihydrolipoic acid.
Substitution: this compound can undergo substitution reactions with various reagents to form different derivatives.
Major Products: The major products formed from these reactions include dihydrolipoic acid and various substituted derivatives of this compound .
Scientific Research Applications
Comparison with Similar Compounds
Lipoamide: A derivative of thioctic acid, used as a cofactor in enzymatic reactions.
Asparagusic Acid: Another organosulfur compound with similar antioxidant properties.
Uniqueness: this compound is unique due to its dual solubility in both fat and water, making it a versatile antioxidant. Its ability to act as a cofactor for multiple enzyme complexes and its potent antioxidant properties distinguish it from other similar compounds .
Properties
IUPAC Name |
5-(dithiolan-3-yl)pentanoic acid | |
---|---|---|
Source | PubChem | |
URL | https://pubchem.ncbi.nlm.nih.gov | |
Description | Data deposited in or computed by PubChem | |
InChI |
InChI=1S/C8H14O2S2/c9-8(10)4-2-1-3-7-5-6-11-12-7/h7H,1-6H2,(H,9,10) | |
Source | PubChem | |
URL | https://pubchem.ncbi.nlm.nih.gov | |
Description | Data deposited in or computed by PubChem | |
InChI Key |
AGBQKNBQESQNJD-UHFFFAOYSA-N | |
Source | PubChem | |
URL | https://pubchem.ncbi.nlm.nih.gov | |
Description | Data deposited in or computed by PubChem | |
Canonical SMILES |
C1CSSC1CCCCC(=O)O | |
Source | PubChem | |
URL | https://pubchem.ncbi.nlm.nih.gov | |
Description | Data deposited in or computed by PubChem | |
Molecular Formula |
C8H14O2S2 | |
Source | PubChem | |
URL | https://pubchem.ncbi.nlm.nih.gov | |
Description | Data deposited in or computed by PubChem | |
DSSTOX Substance ID |
DTXSID7025508 | |
Record name | (+-)-Lipoic acid | |
Source | EPA DSSTox | |
URL | https://comptox.epa.gov/dashboard/DTXSID7025508 | |
Description | DSSTox provides a high quality public chemistry resource for supporting improved predictive toxicology. | |
Molecular Weight |
206.3 g/mol | |
Source | PubChem | |
URL | https://pubchem.ncbi.nlm.nih.gov | |
Description | Data deposited in or computed by PubChem | |
Solubility |
Yellow powder; solubility in ethanol: 50 mg/mL /Synthetic, oxidized form/, Soluble in methanol, ethanol, diethyl ether and chloroform | |
Record name | alpha-Lipoic acid | |
Source | Hazardous Substances Data Bank (HSDB) | |
URL | https://pubchem.ncbi.nlm.nih.gov/source/hsdb/7818 | |
Description | The Hazardous Substances Data Bank (HSDB) is a toxicology database that focuses on the toxicology of potentially hazardous chemicals. It provides information on human exposure, industrial hygiene, emergency handling procedures, environmental fate, regulatory requirements, nanomaterials, and related areas. The information in HSDB has been assessed by a Scientific Review Panel. | |
Mechanism of Action |
Alpha-lipoic acid (LA) shows a protective effect on oxidative stress-induced apoptosis while it induces apoptosis in various cancer cells. Intracellular Ca(2+) plays a central role in triggering apoptotic pathways. In the present study, we aim to investigate whether LA induces apoptosis in lung cancer cells and whether Ca(2+) is involved in LA-induced apoptosis. We found that LA decreased cell viability and increased DNA fragmentation of the cells. LA activated the caspase-independent pathway, determined by upregulation of poly(ADP-ribose) polymerase (PARP) and increased the nuclear level of apoptosis-inducing factor and caspase-dependent apoptotic pathway, determined by increased levels of cytochrome c and PARP-1 cleavage product. LA-induced apoptotic alterations were inhibited in the cells treated with Ca(2+) chelator BAPTA-AM. In conclusion, LA induces apoptosis through caspase-independent and caspase-dependent pathways, which is mediated by intracellular Ca(2+)., Alpha-lipoic acid is known to increase insulin sensitivity in vivo and to stimulate glucose uptake into adipose and muscle cells in vitro. In this study, alpha-lipoic acid was demonstrated to stimulate the autophosphorylation of insulin receptor and glucose uptake into 3T3-L1 adipocytes by reducing the thiol reactivity of intracellular proteins. To elucidate mechanism of this effect, role of protein thiol groups and H(2)O(2) in insulin receptor autophosphorylation and glucose uptake was investigated in 3T3-L1 adipocytes following stimulation with alpha-lipoic acid. Alpha-lipoic acid or insulin treatment of adipocytes increased intracellular level of oxidants, decreased thiol reactivity of the insulin receptor beta-subunit, increased tyrosine phosphorylation of the insulin receptor, and enhanced glucose uptake. Alpha-lipoic acid or insulin-stimulated glucose uptake was inhibited (i) by alkylation of intracellular, but not extracellular, thiol groups downstream of insulin receptor activation, and (ii) by diphenylene iodonium at the level of the insulin receptor autophosphorylation. alpha-Lipoic acid also inhibited protein tyrosine phosphatase activity and decreased thiol reactivity of protein tyrosine phosphatase 1B. These findings indicate that oxidants produced by alpha-lipoic acid or insulin are involved in activation of insulin receptor and in inactivation of protein tyrosine phosphatases, which eventually result in elevated glucose uptake into 3T3-L1 adipocytes., Reactive oxygen (ROS) and nitrogen oxide (RNOS) species are produced as by-products of oxidative metabolism. A major function for ROS and RNOS is immunological host defense. Recent evidence indicate that ROS and RNOS may also function as signaling molecules. However, high levels of ROS and RNOS have been considered to potentially damage cellular macromolecules and have been implicated in the pathogenesis and progression of various chronic diseases. alpha-Lipoic acid and dihydrolipoic acid exhibit direct free radical scavenging properties and as a redox couple, with a low redox potential of -0.32 V, is a strong reductant. Several studies provided evidence that alpha-lipoic acid supplementation decreases oxidative stress and restores reduced levels of other antioxidants in vivo. However, there is also evidence indicating that alpha-lipoic acid and dihydrolipoic acid may exert prooxidant properties in vitro. alpha-Lipoic acid and dihydrolipoic acid were shown to promote the mitochondrial permeability transition in permeabilized hepatocytes and isolated rat liver mitochondria. Dihydrolipoic acid also stimulated superoxide anion production in rat liver mitochondria and submitochondrial particles. alpha-Lipoic acid was recently shown to stimulate glucose uptake into 3T3-L1 adipocytes by increasing intracellular oxidant levels and/or facilitating insulin receptor autophosphorylation presumably by oxidation of critical thiol groups present in the insulin receptor beta-subunit. Whether alpha-lipoic acid and/or dihydrolipoic acid-induced oxidative protein modifications contribute to their versatile effects observed in vivo warrants further investigation., This study investigated the effect of alpha-lipoic acid (ALA) in concentration range 0.7-5.0 mM on the intracellular level of reduced glutathione, the cell cycle phase distribution, the structure of microfilaments and microtubules of normal (3T3) and transformed (3T3-SV40) fibroblasts. We obtained that ALA increased the glutathione content in transformed cells, but did not change its level in normal cells, induced cell cycle arrest of 3T3 cells (but not 3T3-SV40 cells), and disrupted actin microfilaments in cells of both lines. The effect of ALA was compared with N-acetylcysteine (NAC) action. The whole complex of findings allows us to affirm that each of these antioxidants acts on its own target molecules in normal and transformed cells and activates different signal and metabolic pathways in these cells. But at the same time the intermediate steps of ALA and NAC action can be common (alteration of the intracellular level of glutathione, reorganization of actin cytoskeleton, etc.)., For more Mechanism of Action (Complete) data for alpha-Lipoic acid (7 total), please visit the HSDB record page. | |
Record name | alpha-Lipoic acid | |
Source | Hazardous Substances Data Bank (HSDB) | |
URL | https://pubchem.ncbi.nlm.nih.gov/source/hsdb/7818 | |
Description | The Hazardous Substances Data Bank (HSDB) is a toxicology database that focuses on the toxicology of potentially hazardous chemicals. It provides information on human exposure, industrial hygiene, emergency handling procedures, environmental fate, regulatory requirements, nanomaterials, and related areas. The information in HSDB has been assessed by a Scientific Review Panel. | |
Color/Form |
Forms yellowish flakes | |
CAS No. |
1077-28-7, 62-46-4 | |
Record name | (±)-Lipoic acid | |
Source | CAS Common Chemistry | |
URL | https://commonchemistry.cas.org/detail?cas_rn=1077-28-7 | |
Description | CAS Common Chemistry is an open community resource for accessing chemical information. Nearly 500,000 chemical substances from CAS REGISTRY cover areas of community interest, including common and frequently regulated chemicals, and those relevant to high school and undergraduate chemistry classes. This chemical information, curated by our expert scientists, is provided in alignment with our mission as a division of the American Chemical Society. | |
Explanation | The data from CAS Common Chemistry is provided under a CC-BY-NC 4.0 license, unless otherwise stated. | |
Record name | Thioctic acid [INN:BAN:JAN] | |
Source | ChemIDplus | |
URL | https://pubchem.ncbi.nlm.nih.gov/substance/?source=chemidplus&sourceid=0001077287 | |
Description | ChemIDplus is a free, web search system that provides access to the structure and nomenclature authority files used for the identification of chemical substances cited in National Library of Medicine (NLM) databases, including the TOXNET system. | |
Record name | thioctic acid | |
Source | DTP/NCI | |
URL | https://dtp.cancer.gov/dtpstandard/servlet/dwindex?searchtype=NSC&outputformat=html&searchlist=758651 | |
Description | The NCI Development Therapeutics Program (DTP) provides services and resources to the academic and private-sector research communities worldwide to facilitate the discovery and development of new cancer therapeutic agents. | |
Explanation | Unless otherwise indicated, all text within NCI products is free of copyright and may be reused without our permission. Credit the National Cancer Institute as the source. | |
Record name | dl-Thioctic acid | |
Source | DTP/NCI | |
URL | https://dtp.cancer.gov/dtpstandard/servlet/dwindex?searchtype=NSC&outputformat=html&searchlist=90788 | |
Description | The NCI Development Therapeutics Program (DTP) provides services and resources to the academic and private-sector research communities worldwide to facilitate the discovery and development of new cancer therapeutic agents. | |
Explanation | Unless otherwise indicated, all text within NCI products is free of copyright and may be reused without our permission. Credit the National Cancer Institute as the source. | |
Record name | (+-)-Lipoic acid | |
Source | EPA DSSTox | |
URL | https://comptox.epa.gov/dashboard/DTXSID7025508 | |
Description | DSSTox provides a high quality public chemistry resource for supporting improved predictive toxicology. | |
Record name | 5-(dithiolan-3-yl)valeric acid | |
Source | European Chemicals Agency (ECHA) | |
URL | https://echa.europa.eu/substance-information/-/substanceinfo/100.012.793 | |
Description | The European Chemicals Agency (ECHA) is an agency of the European Union which is the driving force among regulatory authorities in implementing the EU's groundbreaking chemicals legislation for the benefit of human health and the environment as well as for innovation and competitiveness. | |
Explanation | Use of the information, documents and data from the ECHA website is subject to the terms and conditions of this Legal Notice, and subject to other binding limitations provided for under applicable law, the information, documents and data made available on the ECHA website may be reproduced, distributed and/or used, totally or in part, for non-commercial purposes provided that ECHA is acknowledged as the source: "Source: European Chemicals Agency, http://echa.europa.eu/". Such acknowledgement must be included in each copy of the material. ECHA permits and encourages organisations and individuals to create links to the ECHA website under the following cumulative conditions: Links can only be made to webpages that provide a link to the Legal Notice page. | |
Record name | 5-(1,2-dithiolan-3-yl)valeric acid | |
Source | European Chemicals Agency (ECHA) | |
URL | https://echa.europa.eu/substance-information/-/substanceinfo/100.000.486 | |
Description | The European Chemicals Agency (ECHA) is an agency of the European Union which is the driving force among regulatory authorities in implementing the EU's groundbreaking chemicals legislation for the benefit of human health and the environment as well as for innovation and competitiveness. | |
Explanation | Use of the information, documents and data from the ECHA website is subject to the terms and conditions of this Legal Notice, and subject to other binding limitations provided for under applicable law, the information, documents and data made available on the ECHA website may be reproduced, distributed and/or used, totally or in part, for non-commercial purposes provided that ECHA is acknowledged as the source: "Source: European Chemicals Agency, http://echa.europa.eu/". Such acknowledgement must be included in each copy of the material. ECHA permits and encourages organisations and individuals to create links to the ECHA website under the following cumulative conditions: Links can only be made to webpages that provide a link to the Legal Notice page. | |
Record name | ALPHA LIPOIC ACID | |
Source | FDA Global Substance Registration System (GSRS) | |
URL | https://gsrs.ncats.nih.gov/ginas/app/beta/substances/73Y7P0K73Y | |
Description | The FDA Global Substance Registration System (GSRS) enables the efficient and accurate exchange of information on what substances are in regulated products. Instead of relying on names, which vary across regulatory domains, countries, and regions, the GSRS knowledge base makes it possible for substances to be defined by standardized, scientific descriptions. | |
Explanation | Unless otherwise noted, the contents of the FDA website (www.fda.gov), both text and graphics, are not copyrighted. They are in the public domain and may be republished, reprinted and otherwise used freely by anyone without the need to obtain permission from FDA. Credit to the U.S. Food and Drug Administration as the source is appreciated but not required. | |
Record name | alpha-Lipoic acid | |
Source | Hazardous Substances Data Bank (HSDB) | |
URL | https://pubchem.ncbi.nlm.nih.gov/source/hsdb/7818 | |
Description | The Hazardous Substances Data Bank (HSDB) is a toxicology database that focuses on the toxicology of potentially hazardous chemicals. It provides information on human exposure, industrial hygiene, emergency handling procedures, environmental fate, regulatory requirements, nanomaterials, and related areas. The information in HSDB has been assessed by a Scientific Review Panel. | |
Melting Point |
47.5-48 °C | |
Record name | alpha-Lipoic acid | |
Source | Hazardous Substances Data Bank (HSDB) | |
URL | https://pubchem.ncbi.nlm.nih.gov/source/hsdb/7818 | |
Description | The Hazardous Substances Data Bank (HSDB) is a toxicology database that focuses on the toxicology of potentially hazardous chemicals. It provides information on human exposure, industrial hygiene, emergency handling procedures, environmental fate, regulatory requirements, nanomaterials, and related areas. The information in HSDB has been assessed by a Scientific Review Panel. | |
Synthesis routes and methods I
Procedure details
Synthesis routes and methods II
Procedure details
Synthesis routes and methods III
Procedure details
Retrosynthesis Analysis
AI-Powered Synthesis Planning: Our tool employs the Template_relevance Pistachio, Template_relevance Bkms_metabolic, Template_relevance Pistachio_ringbreaker, Template_relevance Reaxys, Template_relevance Reaxys_biocatalysis model, leveraging a vast database of chemical reactions to predict feasible synthetic routes.
One-Step Synthesis Focus: Specifically designed for one-step synthesis, it provides concise and direct routes for your target compounds, streamlining the synthesis process.
Accurate Predictions: Utilizing the extensive PISTACHIO, BKMS_METABOLIC, PISTACHIO_RINGBREAKER, REAXYS, REAXYS_BIOCATALYSIS database, our tool offers high-accuracy predictions, reflecting the latest in chemical research and data.
Strategy Settings
Precursor scoring | Relevance Heuristic |
---|---|
Min. plausibility | 0.01 |
Model | Template_relevance |
Template Set | Pistachio/Bkms_metabolic/Pistachio_ringbreaker/Reaxys/Reaxys_biocatalysis |
Top-N result to add to graph | 6 |
Feasible Synthetic Routes
Q1: How does thioctic acid exert its antioxidant effects?
A1: this compound exists in both oxidized (this compound) and reduced (dihydrolipoic acid) forms, both exhibiting antioxidant activity. [] It neutralizes free radicals in both fatty and watery cellular environments, enhancing the activity of other antioxidants like ascorbate and α-tocopherol by regenerating them from their radical forms. []
Q2: What is the role of this compound in glucose metabolism?
A2: this compound improves insulin-responsive glucose utilization. [] Studies show it stimulates glucose uptake in muscle cells and adipocytes, comparable to insulin. This effect involves the activation of phosphatidylinositol 3-kinase and leads to the redistribution of GLUT1 and GLUT4 glucose transporters. []
Q3: What is the molecular structure of this compound?
A3: this compound (1,2-dithiolane-3-pentanoic acid) has a chiral center and exists as two enantiomers: (+)-thioctic acid (R-enantiomer) and (−)-thioctic acid (S-enantiomer). The naturally occurring form is the (+)-enantiomer. [, ]
Q4: Are there differences in the biological activity of this compound enantiomers?
A4: Research suggests that the (+)-enantiomer of this compound exhibits more pronounced biological activity compared to the (−)-enantiomer and racemic mixture in several contexts, including pain relief, neuroprotection, and antioxidant effects. [, , , , ]
Q5: What challenges are associated with the formulation of this compound into solid dosage forms?
A5: this compound presents challenges in tabletting due to its poor flowability, low melting point, and potential for sintering during drying. [] Adhesion to pressing tools can also lead to uneven tablet surfaces. []
Q6: How can the stability and bioavailability of this compound be improved in pharmaceutical formulations?
A6: Strategies include using specific granulometric compositions of this compound, incorporating basic excipients, and utilizing glidants to improve flowability. [] Solid dispersions using carriers like polyvinylpyrrolidone (PVP) and hydroxypropyl methylcellulose (HPMC) have shown enhanced dissolution rates, potentially leading to improved bioavailability. [] Liposomal encapsulation also shows promise for enhancing chemical stability and targeted delivery. []
Q7: How does the dissolution rate of this compound vary among different formulations?
A7: Dissolution studies of multisource this compound tablets revealed significant differences in the rate and degree of active ingredient release. [] These variations highlight the importance of formulation and manufacturing processes in achieving desired dissolution profiles. []
Q8: What in vitro models are used to study the effects of this compound?
A8: Researchers utilize various cell lines, including rat pheochromocytoma PC12 cells, to investigate the antioxidant activity of this compound against hydrogen peroxide-induced apoptosis and oxidative stress. []
Q9: What animal models are used to study the effects of this compound?
A9: Spontaneously hypertensive rats (SHR) serve as an established model for studying hypertension and oxidative stress-related organ damage. [, , , , ] Researchers also employ models like sciatic nerve ligation in rats to investigate the neuroprotective potential of this compound in peripheral neuropathies. [, ]
Q10: What clinical evidence supports the use of this compound in diabetic neuropathy?
A10: Clinical trials have demonstrated the efficacy of this compound in improving symptoms of diabetic peripheral neuropathy. [, , ] Studies suggest that this compound can improve nerve conduction velocity, reduce neuropathic pain, and enhance quality of life in patients with diabetic neuropathy. [, ]
Q11: What is known about the toxicity profile of this compound enantiomers?
A11: Preclinical studies in rats indicate potential toxicity differences between this compound enantiomers. [] Subchronic administration of the (−)-enantiomer led to more pronounced organ toxicity signs and enhanced caspase-3 activity in certain organs compared to the (+)-enantiomer and racemate. []
Q12: Are there reported cases of this compound-induced liver toxicity?
A12: Although considered a potential hepatoprotectant, a case report documented acute cholestatic hepatitis potentially linked to this compound use. [] While rare, this case highlights the importance of monitoring liver function in patients using this compound. []
Q13: What are the prospects for targeted delivery of this compound?
A13: Liposomal encapsulation of this compound conjugates shows promise for targeted delivery to specific sites, potentially improving therapeutic efficacy and minimizing off-target effects. []
Disclaimer and Information on In-Vitro Research Products
Please be aware that all articles and product information presented on BenchChem are intended solely for informational purposes. The products available for purchase on BenchChem are specifically designed for in-vitro studies, which are conducted outside of living organisms. In-vitro studies, derived from the Latin term "in glass," involve experiments performed in controlled laboratory settings using cells or tissues. It is important to note that these products are not categorized as medicines or drugs, and they have not received approval from the FDA for the prevention, treatment, or cure of any medical condition, ailment, or disease. We must emphasize that any form of bodily introduction of these products into humans or animals is strictly prohibited by law. It is essential to adhere to these guidelines to ensure compliance with legal and ethical standards in research and experimentation.