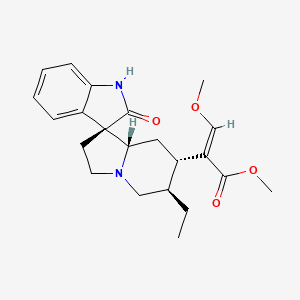
Isorhynchophylline
Overview
Description
This compound has been extensively used for its pharmacological properties, including antihypertensive, neuroprotective, and anti-inflammatory effects . It is one of the major active components of Uncaria rhynchophylla and has shown potential in treating various ailments such as hypertension, stroke, and Alzheimer’s disease .
Mechanism of Action
Target of Action
Isorhynchophylline (IRN) is an active pharmacological component of Uncaria rhynchophylla . It primarily targets vascular endothelial cells and N-methyl-D-aspartic acid (NMDA) receptors . These targets play crucial roles in maintaining vascular health and regulating emotional and cognitive processes .
Mode of Action
IRN interacts with its targets to exert a variety of effects. It provides protection to vascular endothelial cells, exhibits anti-inflammatory, anti-thrombotic, and anti-smooth muscle cell proliferation properties . In the context of NMDA receptors, IRN modulates these receptors, which play an important role in emotion and memory processes .
Biochemical Pathways
IRN affects several metabolic pathways. According to a study, three metabolic pathways were identified to be involved in IRN’s action: glycerophospholipid metabolism, linoleic acid metabolism, and alpha-linolenic acid metabolism . These pathways are crucial for lipid metabolism and inflammation, which are key factors in conditions like atherosclerosis .
Pharmacokinetics
The pharmacokinetics of IRN involve its absorption, distribution, metabolism, and excretion (ADME). After oral administration, IRN is metabolized to form hydroxylated metabolites . It has been reported that a significant portion of IRN is excreted into the feces and urine of rats after oral administration
Result of Action
The action of IRN results in several molecular and cellular effects. It has been found to improve corticosterone-induced in vivo long-term potentiation (LTP) impairment, indicating positive effects on stress . Moreover, it has been shown to ameliorate stress-induced emotional disorders and cognitive impairment . In the context of atherosclerosis, IRN has been found to have a protective effect on vascular endothelial cells and exhibit anti-inflammatory, anti-thrombotic, and anti-smooth muscle cell proliferation properties .
Biochemical Analysis
Biochemical Properties
Isorhynchophylline plays a significant role in various biochemical reactions. It interacts with several enzymes, proteins, and other biomolecules. For instance, this compound has been shown to inhibit the activity of monoamine oxidase A (MAO-A), an enzyme involved in the degradation of neurotransmitters . Additionally, it modulates the activity of caspase-3, an enzyme crucial for the execution phase of apoptosis . This compound also interacts with proteins involved in cell signaling pathways, such as the PI3K/Akt pathway, which plays a role in cell survival and proliferation .
Cellular Effects
This compound exerts various effects on different types of cells and cellular processes. In neuronal cells, it has been shown to protect against beta-amyloid-induced neurotoxicity by reducing oxidative stress and stabilizing mitochondrial membrane potential . In cancer cells, this compound induces apoptosis and inhibits cell proliferation, migration, and invasion . It also affects cell signaling pathways, such as the MAPK pathway, which is involved in cell growth and differentiation . Furthermore, this compound influences gene expression by modulating the levels of pro-apoptotic and anti-apoptotic proteins .
Molecular Mechanism
The molecular mechanism of this compound involves several key interactions at the molecular level. It binds to and inhibits the activity of monoamine oxidase A, leading to increased levels of neurotransmitters such as norepinephrine and serotonin . This compound also activates the PI3K/Akt pathway, promoting cell survival and proliferation . Additionally, it inhibits the MAPK pathway, reducing cell growth and differentiation . These interactions result in changes in gene expression, including the upregulation of anti-apoptotic proteins and the downregulation of pro-apoptotic proteins .
Temporal Effects in Laboratory Settings
In laboratory settings, the effects of this compound have been observed to change over time. Studies have shown that this compound remains stable under various conditions, with minimal degradation over time . Long-term exposure to this compound has been associated with sustained neuroprotective effects, including the reduction of oxidative stress and the stabilization of mitochondrial membrane potential . In cancer cells, prolonged treatment with this compound leads to sustained inhibition of cell proliferation and induction of apoptosis .
Dosage Effects in Animal Models
The effects of this compound vary with different dosages in animal models. In mice, intragastric administration of this compound at doses of 10, 20, and 40 mg/kg for seven days resulted in a significant reduction of immobility time in forced swimming and tail suspension tests, indicating antidepressant-like effects . Higher doses of this compound have been associated with increased neuroprotective and anti-inflammatory effects . At very high doses, this compound may exhibit toxic effects, including hepatotoxicity and nephrotoxicity .
Metabolic Pathways
This compound is involved in several metabolic pathways. It has been shown to interact with enzymes involved in glycerophospholipid metabolism, linoleic acid metabolism, and alpha-linolenic acid metabolism . These interactions result in changes in metabolic flux and metabolite levels, contributing to the pharmacological effects of this compound. Additionally, this compound affects the levels of neurotransmitters and other metabolites in the brain, further influencing its neuroprotective and antidepressant-like effects .
Transport and Distribution
This compound is transported and distributed within cells and tissues through various mechanisms. It interacts with transporters and binding proteins that facilitate its uptake and distribution . In the liver, this compound is transported via specific transporters and is distributed to various tissues, including the brain, where it exerts its neuroprotective effects . The localization and accumulation of this compound within cells are influenced by its interactions with cellular transporters and binding proteins .
Subcellular Localization
The subcellular localization of this compound plays a crucial role in its activity and function. This compound has been shown to localize to the mitochondria, where it stabilizes mitochondrial membrane potential and reduces oxidative stress . It also localizes to the cytoplasm and interacts with various signaling proteins, influencing cell signaling pathways and gene expression . The targeting of this compound to specific subcellular compartments is mediated by post-translational modifications and targeting signals .
Preparation Methods
Synthetic Routes and Reaction Conditions: The synthesis of isorhynchophylline involves several steps, including the formation of key intermediates through one-pot transformations. A notable synthetic route includes the preparation of tetracyclic intermediates via N-alkylation and cross-dehydrogenative coupling sequences . These intermediates are then subjected to further transformations to yield this compound.
Industrial Production Methods: Industrial production of this compound typically involves the extraction of the compound from Uncaria rhynchophylla using solvents such as ethanol or methanol. The extract is then purified using chromatographic techniques to isolate this compound . This method ensures high purity and yield of the compound.
Chemical Reactions Analysis
Types of Reactions: Isorhynchophylline undergoes various chemical reactions, including oxidation, reduction, and substitution. These reactions are essential for modifying the compound’s structure and enhancing its pharmacological properties .
Common Reagents and Conditions:
Oxidation: Common oxidizing agents such as potassium permanganate or hydrogen peroxide are used under controlled conditions to oxidize this compound.
Reduction: Reducing agents like sodium borohydride or lithium aluminum hydride are employed to reduce specific functional groups within the compound.
Substitution: Nucleophilic substitution reactions are carried out using reagents like sodium hydroxide or potassium carbonate.
Major Products: The major products formed from these reactions include various derivatives of this compound with altered pharmacological properties. These derivatives are often studied for their enhanced therapeutic effects .
Scientific Research Applications
Isorhynchophylline has a wide range of scientific research applications:
Chemistry: It is used as a precursor for synthesizing other bioactive compounds and studying reaction mechanisms.
Medicine: this compound has demonstrated potential in treating conditions such as hypertension, Alzheimer’s disease, and cancer.
Comparison with Similar Compounds
Isorhynchophylline is often compared with other oxindole alkaloids such as rhynchophylline, mitraphylline, and formosanine . While these compounds share similar structures, this compound is unique in its potent antihypertensive and neuroprotective effects . The following table highlights the differences:
Compound | Unique Properties |
---|---|
This compound | Potent antihypertensive and neuroprotective effects |
Rhynchophylline | Similar structure but less potent in neuroprotection |
Mitraphylline | Known for its anti-inflammatory properties |
Formosanine | Exhibits moderate antihypertensive effects |
Properties
IUPAC Name |
methyl (E)-2-[(3S,6'R,7'S,8'aS)-6'-ethyl-2-oxospiro[1H-indole-3,1'-3,5,6,7,8,8a-hexahydro-2H-indolizine]-7'-yl]-3-methoxyprop-2-enoate | |
---|---|---|
Source | PubChem | |
URL | https://pubchem.ncbi.nlm.nih.gov | |
Description | Data deposited in or computed by PubChem | |
InChI |
InChI=1S/C22H28N2O4/c1-4-14-12-24-10-9-22(17-7-5-6-8-18(17)23-21(22)26)19(24)11-15(14)16(13-27-2)20(25)28-3/h5-8,13-15,19H,4,9-12H2,1-3H3,(H,23,26)/b16-13+/t14-,15-,19-,22-/m0/s1 | |
Source | PubChem | |
URL | https://pubchem.ncbi.nlm.nih.gov | |
Description | Data deposited in or computed by PubChem | |
InChI Key |
DAXYUDFNWXHGBE-VKCGGMIFSA-N | |
Source | PubChem | |
URL | https://pubchem.ncbi.nlm.nih.gov | |
Description | Data deposited in or computed by PubChem | |
Canonical SMILES |
CCC1CN2CCC3(C2CC1C(=COC)C(=O)OC)C4=CC=CC=C4NC3=O | |
Source | PubChem | |
URL | https://pubchem.ncbi.nlm.nih.gov | |
Description | Data deposited in or computed by PubChem | |
Isomeric SMILES |
CC[C@H]1CN2CC[C@@]3([C@@H]2C[C@@H]1/C(=C\OC)/C(=O)OC)C4=CC=CC=C4NC3=O | |
Source | PubChem | |
URL | https://pubchem.ncbi.nlm.nih.gov | |
Description | Data deposited in or computed by PubChem | |
Molecular Formula |
C22H28N2O4 | |
Source | PubChem | |
URL | https://pubchem.ncbi.nlm.nih.gov | |
Description | Data deposited in or computed by PubChem | |
Molecular Weight |
384.5 g/mol | |
Source | PubChem | |
URL | https://pubchem.ncbi.nlm.nih.gov | |
Description | Data deposited in or computed by PubChem | |
CAS No. |
6859-01-4 | |
Record name | 7-Isorhyncophylline | |
Source | CAS Common Chemistry | |
URL | https://commonchemistry.cas.org/detail?cas_rn=6859-01-4 | |
Description | CAS Common Chemistry is an open community resource for accessing chemical information. Nearly 500,000 chemical substances from CAS REGISTRY cover areas of community interest, including common and frequently regulated chemicals, and those relevant to high school and undergraduate chemistry classes. This chemical information, curated by our expert scientists, is provided in alignment with our mission as a division of the American Chemical Society. | |
Explanation | The data from CAS Common Chemistry is provided under a CC-BY-NC 4.0 license, unless otherwise stated. | |
Record name | 7-Isorhyncophylline | |
Source | ChemIDplus | |
URL | https://pubchem.ncbi.nlm.nih.gov/substance/?source=chemidplus&sourceid=0006859014 | |
Description | ChemIDplus is a free, web search system that provides access to the structure and nomenclature authority files used for the identification of chemical substances cited in National Library of Medicine (NLM) databases, including the TOXNET system. | |
Record name | 6859-01-4 | |
Source | European Chemicals Agency (ECHA) | |
URL | https://echa.europa.eu/information-on-chemicals | |
Description | The European Chemicals Agency (ECHA) is an agency of the European Union which is the driving force among regulatory authorities in implementing the EU's groundbreaking chemicals legislation for the benefit of human health and the environment as well as for innovation and competitiveness. | |
Explanation | Use of the information, documents and data from the ECHA website is subject to the terms and conditions of this Legal Notice, and subject to other binding limitations provided for under applicable law, the information, documents and data made available on the ECHA website may be reproduced, distributed and/or used, totally or in part, for non-commercial purposes provided that ECHA is acknowledged as the source: "Source: European Chemicals Agency, http://echa.europa.eu/". Such acknowledgement must be included in each copy of the material. ECHA permits and encourages organisations and individuals to create links to the ECHA website under the following cumulative conditions: Links can only be made to webpages that provide a link to the Legal Notice page. | |
Record name | ISORHYNCHOPHYLLINE | |
Source | FDA Global Substance Registration System (GSRS) | |
URL | https://gsrs.ncats.nih.gov/ginas/app/beta/substances/7F4P99KHLJ | |
Description | The FDA Global Substance Registration System (GSRS) enables the efficient and accurate exchange of information on what substances are in regulated products. Instead of relying on names, which vary across regulatory domains, countries, and regions, the GSRS knowledge base makes it possible for substances to be defined by standardized, scientific descriptions. | |
Explanation | Unless otherwise noted, the contents of the FDA website (www.fda.gov), both text and graphics, are not copyrighted. They are in the public domain and may be republished, reprinted and otherwise used freely by anyone without the need to obtain permission from FDA. Credit to the U.S. Food and Drug Administration as the source is appreciated but not required. | |
Retrosynthesis Analysis
AI-Powered Synthesis Planning: Our tool employs the Template_relevance Pistachio, Template_relevance Bkms_metabolic, Template_relevance Pistachio_ringbreaker, Template_relevance Reaxys, Template_relevance Reaxys_biocatalysis model, leveraging a vast database of chemical reactions to predict feasible synthetic routes.
One-Step Synthesis Focus: Specifically designed for one-step synthesis, it provides concise and direct routes for your target compounds, streamlining the synthesis process.
Accurate Predictions: Utilizing the extensive PISTACHIO, BKMS_METABOLIC, PISTACHIO_RINGBREAKER, REAXYS, REAXYS_BIOCATALYSIS database, our tool offers high-accuracy predictions, reflecting the latest in chemical research and data.
Strategy Settings
Precursor scoring | Relevance Heuristic |
---|---|
Min. plausibility | 0.01 |
Model | Template_relevance |
Template Set | Pistachio/Bkms_metabolic/Pistachio_ringbreaker/Reaxys/Reaxys_biocatalysis |
Top-N result to add to graph | 6 |
Feasible Synthetic Routes
Disclaimer and Information on In-Vitro Research Products
Please be aware that all articles and product information presented on BenchChem are intended solely for informational purposes. The products available for purchase on BenchChem are specifically designed for in-vitro studies, which are conducted outside of living organisms. In-vitro studies, derived from the Latin term "in glass," involve experiments performed in controlled laboratory settings using cells or tissues. It is important to note that these products are not categorized as medicines or drugs, and they have not received approval from the FDA for the prevention, treatment, or cure of any medical condition, ailment, or disease. We must emphasize that any form of bodily introduction of these products into humans or animals is strictly prohibited by law. It is essential to adhere to these guidelines to ensure compliance with legal and ethical standards in research and experimentation.