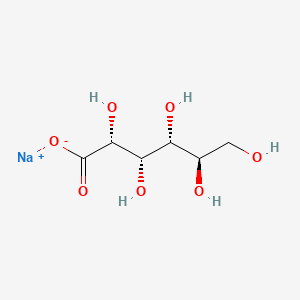
Sodium gluconate
Overview
Description
Sodium gluconate is the sodium salt of gluconic acid, with the chemical formula C6H11NaO7. It is a white, water-soluble powder known for its excellent chelating properties, particularly in alkaline and concentrated alkaline solutions. This compound is non-toxic, biodegradable, and finds applications across various industries, including textiles, metal surface treatment, and cement .
Mechanism of Action
Target of Action
Sodium gluconate, the sodium salt of gluconic acid , is known for its chelating properties . It primarily targets metal ions in various applications, forming stable complexes with them . In the context of cement and concrete, it interacts with the calcium ions (Ca2+) present .
Mode of Action
The mode of action of this compound involves a chemical reaction where gluconic acid is neutralized with sodium hydroxide (NaOH). This reaction results in the formation of this compound, where the sodium ions (Na+) replace the hydrogen ions (H+) in gluconic acid . In the context of cement, this compound acts as a set-retarder but usually improves the flow properties of cement pastes at constant water-to-cement ratios .
Biochemical Pathways
This compound is involved in the metabolism of glucose. Glucose, or other sugar sources, serve as the substrate for microorganisms, typically bacteria or fungi, to produce gluconic acid . This gluconic acid is then converted into this compound . In humans, gluconate metabolism involves the enzyme gluconokinase, which converts D-gluconate to 6-phospho-D-gluconate .
Pharmacokinetics
It’s also likely to be metabolized and excreted efficiently due to its involvement in common metabolic pathways .
Result of Action
The result of this compound’s action depends on its application. In the context of cement, it improves the workability and performance of concrete . In other applications, it forms stable complexes with metal ions, which can be useful in industries such as textile and metal surface treatment .
Action Environment
This compound is known for its biodegradability, which means it can break down naturally in the environment . It is considered environmentally friendly . Its action, efficacy, and stability can be influenced by environmental factors such as pH and temperature . It is generally stable over a wide range of conditions .
Biochemical Analysis
Biochemical Properties
Sodium gluconate plays a significant role in biochemical reactions. It has been found to significantly increase the biomass of certain microorganisms and the expression of most genes involved in biochemical degradation . Activities of central carbon metabolism, fatty acid β-oxidation, and oxidative phosphorylation were all promoted .
Cellular Effects
This compound has been observed to have various effects on cellular processes. For instance, it has been found to stimulate the production of intestinal butyrate . It also acts as a chelating agent, which can influence cell function by binding to metal ions and altering their chemical behavior .
Molecular Mechanism
The molecular mechanism of this compound involves a chemical reaction where gluconic acid is neutralized with sodium hydroxide (NaOH) . This reaction results in the formation of this compound, where the sodium ions (Na+) replace the hydrogen ions (H+) in gluconic acid .
Temporal Effects in Laboratory Settings
In laboratory settings, the effects of this compound can change over time. For instance, this compound has been found to prolong the setting time of certain materials, enhancing workability . Higher dosages led to a longer setting time, increased workability, reduced compressive strength, and improved porosity .
Metabolic Pathways
This compound is involved in several metabolic pathways. For instance, it has been found to promote the intracellular transport of certain compounds, along with the secretion of biosurfactants, enhancing emulsification and solubilization capabilities for improved dissolution and degradation .
Transport and Distribution
This compound is transported and distributed within cells and tissues. Sodium-glucose co-transporters (SGLTs) are responsible for the “secondary-active” transport of glucose and other substrates across cellular membranes . They concentrate glucose inside the cell using electrochemical energy from the transmembrane Na+ gradient, employing an alternating access co-transport mechanism .
Preparation Methods
Synthetic Routes and Reaction Conditions: Sodium gluconate can be synthesized through the fermentation of glucose by certain microorganisms, such as strains of Aspergillus niger or Pseudomonas. The primary product of this fermentation is gluconic acid, which is then neutralized with sodium hydroxide to form this compound .
Industrial Production Methods:
Fermentation Process: Glucose is fermented by microorganisms to produce gluconic acid. This acid is then neutralized with sodium hydroxide to yield this compound.
Chemical Synthesis: Glucose undergoes oxidation in the presence of oxygen and sodium carbonate in water, catalyzed by gold on hydroxyapatite (Au/HAP), to produce this compound.
Chemical Reactions Analysis
Types of Reactions: Sodium gluconate primarily undergoes chelation reactions due to its ability to form stable complexes with metal ions. It does not typically participate in oxidation, reduction, or substitution reactions under standard conditions.
Common Reagents and Conditions:
Neutralization: The conversion of gluconic acid to this compound involves neutralization with sodium hydroxide.
Major Products: The primary product of these reactions is the stable chelate complexes formed with various metal ions.
Scientific Research Applications
Sodium gluconate has a wide range of applications in scientific research and industry:
Comparison with Similar Compounds
Ethylenediaminetetraacetic acid (EDTA): Another widely used chelating agent with strong metal-binding properties.
Citric Acid: A natural chelating agent used in various applications, including food and cleaning products.
Uniqueness of Sodium Gluconate:
Biodegradability: this compound is readily biodegradable, making it environmentally friendly compared to some other chelating agents like EDTA.
Non-toxicity: It is non-toxic and safe for use in food and pharmaceutical applications.
Chelating Efficiency: this compound is particularly effective in alkaline solutions, surpassing many other chelating agents in this respect.
Properties
CAS No. |
527-07-1 |
---|---|
Molecular Formula |
C6H12NaO7 |
Molecular Weight |
219.14 g/mol |
IUPAC Name |
sodium;(2R,3S,4R,5R)-2,3,4,5,6-pentahydroxyhexanoate |
InChI |
InChI=1S/C6H12O7.Na/c7-1-2(8)3(9)4(10)5(11)6(12)13;/h2-5,7-11H,1H2,(H,12,13);/t2-,3-,4+,5-;/m1./s1 |
InChI Key |
IAYLKIVGMOMHDX-JJKGCWMISA-N |
SMILES |
C(C(C(C(C(C(=O)[O-])O)O)O)O)O.[Na+] |
Isomeric SMILES |
C([C@H]([C@H]([C@@H]([C@H](C(=O)O)O)O)O)O)O.[Na] |
Canonical SMILES |
C(C(C(C(C(C(=O)O)O)O)O)O)O.[Na] |
boiling_point |
No boiling point at normal pressure; decomposes at 196-198 °C |
density |
1.8 g/cm³ |
melting_point |
170-175 °C |
14906-97-9 527-07-1 |
|
physical_description |
Liquid; Other Solid; NKRA; Liquid, Other Solid; Dry Powder, Liquid; Dry Powder White to tan, granular to fine, crystalline powder White solid; [ICSC] Technical product may have a pleasant odor; [Merck Index] White crystals with a pleasant odor; [Alfa Aesar MSDS] WHITE CRYSTALS. |
Related CAS |
14906-97-9 |
solubility |
Very soluble in water. Sparingly soluble in ethanol Solubility in water, g/100ml at 25 °C: 59 (good) |
Synonyms |
oron gluconate D-gluconate D-gluconic acid dextronic acid gluconate gluconic acid gluconic acid, (113)indium-labeled gluconic acid, (14)C-labeled gluconic acid, (159)dysprosium-labeled salt gluconic acid, (99)technecium (5+) salt gluconic acid, 1-(14)C-labeled gluconic acid, 6-(14)C-labeled gluconic acid, aluminum (3:1) salt gluconic acid, ammonium salt gluconic acid, calcium salt gluconic acid, cesium(+3) salt gluconic acid, cobalt (2:1) salt gluconic acid, copper salt gluconic acid, Fe(+2) salt, dihydrate gluconic acid, lanthanum(+3) salt gluconic acid, magnesium (2:1) salt gluconic acid, manganese (2:1) salt gluconic acid, monolithium salt gluconic acid, monopotassium salt gluconic acid, monosodium salt gluconic acid, potassium salt gluconic acid, sodium salt gluconic acid, strontium (2:1) salt gluconic acid, tin(+2) salt gluconic acid, zinc salt lithium gluconate magnerot magnesium gluconate maltonic acid manganese gluconate pentahydroxycaproic acid sodium gluconate zinc gluconate |
Origin of Product |
United States |
Retrosynthesis Analysis
AI-Powered Synthesis Planning: Our tool employs the Template_relevance Pistachio, Template_relevance Bkms_metabolic, Template_relevance Pistachio_ringbreaker, Template_relevance Reaxys, Template_relevance Reaxys_biocatalysis model, leveraging a vast database of chemical reactions to predict feasible synthetic routes.
One-Step Synthesis Focus: Specifically designed for one-step synthesis, it provides concise and direct routes for your target compounds, streamlining the synthesis process.
Accurate Predictions: Utilizing the extensive PISTACHIO, BKMS_METABOLIC, PISTACHIO_RINGBREAKER, REAXYS, REAXYS_BIOCATALYSIS database, our tool offers high-accuracy predictions, reflecting the latest in chemical research and data.
Strategy Settings
Precursor scoring | Relevance Heuristic |
---|---|
Min. plausibility | 0.01 |
Model | Template_relevance |
Template Set | Pistachio/Bkms_metabolic/Pistachio_ringbreaker/Reaxys/Reaxys_biocatalysis |
Top-N result to add to graph | 6 |
Feasible Synthetic Routes
Disclaimer and Information on In-Vitro Research Products
Please be aware that all articles and product information presented on BenchChem are intended solely for informational purposes. The products available for purchase on BenchChem are specifically designed for in-vitro studies, which are conducted outside of living organisms. In-vitro studies, derived from the Latin term "in glass," involve experiments performed in controlled laboratory settings using cells or tissues. It is important to note that these products are not categorized as medicines or drugs, and they have not received approval from the FDA for the prevention, treatment, or cure of any medical condition, ailment, or disease. We must emphasize that any form of bodily introduction of these products into humans or animals is strictly prohibited by law. It is essential to adhere to these guidelines to ensure compliance with legal and ethical standards in research and experimentation.