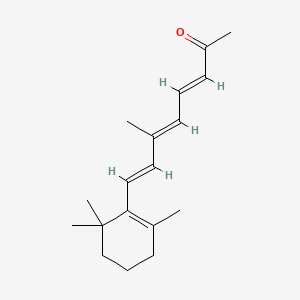
beta-Apo-13-carotenone
Overview
Description
It is postulated to be an early cleavage product of β-carotene en route to trisporic acids, which act as morphogenetic factors during the sexual reproduction of zygomycetes . D’Orenone has been studied for its role in blocking the highly polarized tip growth of root hairs by interfering with the PIN2-mediated auxin transport network in the root apex .
Preparation Methods
D’Orenone is synthesized as an early cleavage product of β-carotene. The synthetic route involves the oxidative cleavage of β-carotene, a process facilitated by carotenoid cleavage dioxygenases (CCDs). This reaction yields various apocarotenoids, including D’Orenone
Chemical Reactions Analysis
Formation via Oxidative Cleavage of β-Carotene
Beta-Apo-13-carotenone is primarily synthesized through the eccentric cleavage of β-carotene at the 13,14 double bond. This process occurs via:
-
Enzymatic pathways : Catalyzed by β-carotene 9',10'-dioxygenase and β-carotene 15,15'-dioxygenase, which generate biradical intermediates during oxygen-dependent cleavage .
-
Non-enzymatic autoxidation : In the presence of triplet oxygen (³O₂), β-carotene undergoes oxidation to form β-carotene endoperoxides (βCar-EPOs), which subsequently degrade into β-Apo-13-carotenone .
Triplet-State Reactivity with Molecular Oxygen
The compound’s triplet excited state (³βCar) drives its reactions with oxygen :
Reaction Type | Mechanism | Products |
---|---|---|
Triplet-triplet quenching | ³βCar + ³O₂ → βCar-EPOs | Endoperoxide intermediates (e.g., βCar-7,10-EPO) |
Photochemical breakdown | Light-induced cleavage of βCar-EPOs | ¹O₂, free radicals, and degraded carotenes (e.g., β-cyclocitral) |
This reactivity explains its pro-oxidant activity , as photolysis of βCar-EPOs releases singlet oxygen (¹O₂) and triggers free radical cascades .
Receptor Binding and Antagonism
This compound interacts with nuclear receptors through competitive binding :
Retinoic Acid Receptor (RAR) Affinity
Receptor Subtype | Binding Affinity (nM) | Comparison to atRA |
---|---|---|
RARα | 5.2 ± 1.3 | 1.4-fold weaker |
RARβ | 4.8 ± 0.9 | Equivalent |
RARγ | 6.7 ± 1.1 | 1.2-fold weaker |
Data derived from competitive radioligand assays .
The compound acts as a high-affinity antagonist for RARs and retinoid X receptor α (RXRα), effectively blocking 9-cis-retinoic acid–mediated transactivation at concentrations as low as 1 nM .
Degradation and Byproduct Formation
This compound undergoes further oxidation and fragmentation:
-
Thermal decomposition : Stable in the dark but degrades into volatile aldehydes (e.g., diacetyl) and low-mass radicals under aerobic conditions .
-
Photolytic breakdown : Releases ¹O₂ and generates allylic biradicals, which fragment into products like apo-10'-carotenal and β-cyclocitral via Norrish type II reactions .
Synthetic Routes
Key laboratory synthesis methods include :
-
Wittig reaction : Using β-ionylideneacetaldehyde and 2-oxopropyltriphenylphosphonium chloride.
-
Oxidative cleavage : Of β-carotene with MnO₂ or other oxidizing agents, yielding a mixture of apocarotenoids.
Biological Implications
-
Antioxidant-prooxidant swap : Photochemical degradation generates free radicals linked to oxidative stress .
-
Gene regulation : Antagonism of RAR/RXR signaling modulates retinoic acid–responsive genes, impacting cellular differentiation and metabolism .
This multifaceted reactivity positions this compound as a critical metabolite influencing both redox balance and nuclear receptor signaling in biological systems.
Scientific Research Applications
Molecular Mechanisms
β-Apo-13-carotenone has been shown to interact with RXRα, a critical nuclear receptor involved in various biological processes, including cell differentiation and metabolism. Research indicates that β-apo-13-carotenone inhibits the transactivation of RXRα without interfering with coactivator binding, suggesting a unique mechanism of action compared to other known antagonists . Specifically, it induces the formation of a transcriptionally silent RXR tetramer, which prevents the activation of target genes by retinoic acid .
Table 1: Molecular Interactions of β-Apo-13-Carotenone
Gene Regulation
β-Apo-13-carotenone's ability to modulate gene expression has been a focal point in studies examining its role in cancer biology. For instance, it has been shown to inhibit the expression of caspase 9 in mammary carcinoma cells, indicating its potential as a therapeutic agent in cancer treatment . This inhibition is significant as caspase 9 is a key player in apoptotic pathways.
Case Study: Inhibition of Caspase 9 Expression
In a study involving MCF-7 breast cancer cells, treatment with β-apo-13-carotenone resulted in a marked reduction in caspase 9 mRNA levels when combined with 9-cis-retinoic acid. This suggests that β-apo-13-carotenone can effectively modulate apoptotic signaling pathways, potentially leading to therapeutic applications in cancer management .
Potential Therapeutic Applications
The unique properties of β-apo-13-carotenone as an RXR antagonist suggest several potential therapeutic applications:
- Cancer Therapy : Its ability to inhibit RXR-mediated gene expression may provide a novel approach to cancer treatment by modulating pathways involved in cell survival and apoptosis.
- Metabolic Disorders : Given RXR's role in metabolism, β-apo-13-carotenone could be explored for its effects on metabolic regulation.
- Nutritional Supplements : As a carotenoid derivative, it may have applications in dietary supplements aimed at improving health outcomes related to vitamin A metabolism.
Mechanism of Action
D’Orenone exerts its effects by interfering with the PIN2-mediated auxin transport network in the root apex. It lowers the auxin concentration in trichoblasts via PIN2-mediated auxin efflux, which is essential for root hair growth . Additionally, D’Orenone functions as an antagonist of the retinoid X receptor alpha (RXRα), competing with 9-cis-retinoic acid for binding and inhibiting its activation .
Comparison with Similar Compounds
D’Orenone is structurally similar to other apocarotenoids derived from the oxidative cleavage of carotenoids. Similar compounds include:
β-Apo-13-carotenone:
Strigolactones: These are plant hormones derived from carotenoids that play a role in shoot branching and root development.
Trisporic acids: These are morphogenetic factors involved in the sexual reproduction of zygomycetes and are derived from the same biosynthetic pathway as D’Orenone.
D’Orenone is unique in its specific role in blocking root hair growth and its potential therapeutic applications as an RXRα antagonist .
Biological Activity
Beta-apo-13-carotenone (β-apo-13-carotenone) is a significant metabolite derived from the eccentric cleavage of β-carotene, a well-known carotenoid. This compound has attracted attention due to its unique biological activities, particularly its role as a modulator of retinoid X receptor (RXR) signaling pathways. This article delves into the biological activity of β-apo-13-carotenone, highlighting its mechanisms of action, effects on cellular processes, and potential implications in health and disease.
β-Apo-13-carotenone functions primarily as an antagonist of RXRα, a nuclear receptor that plays a crucial role in regulating gene expression in response to retinoids. Research indicates that β-apo-13-carotenone can inhibit the activation of RXRα by 9-cis-retinoic acid (9cRA), a naturally occurring ligand. This antagonistic effect has been observed at remarkably low concentrations, with effective inhibition noted at levels as low as 1 nM .
Molecular Interactions
Molecular modeling studies suggest that β-apo-13-carotenone interacts with RXRα similarly to other known antagonists. Specifically, it induces tetramerization of RXRα's ligand-binding domain (LBD), leading to the formation of a transcriptionally inactive complex. This contrasts with other antagonists like UVI3003, which do not induce such oligomerization .
Biological Effects
The biological effects of β-apo-13-carotenone extend beyond RXR modulation. It has been shown to influence various cellular processes:
- Gene Expression Regulation : By antagonizing RXRα, β-apo-13-carotenone alters the expression of genes regulated by retinoids, including those involved in cell differentiation and apoptosis .
- Cellular Uptake and Metabolism : Studies indicate that β-apo-13-carotenone is rapidly taken up by intestinal cells and extensively metabolized, suggesting its bioavailability and potential physiological relevance .
Case Studies and Research Findings
Several studies have investigated the biological activity of β-apo-13-carotenone:
Study 1: RXRα Antagonism
In a study assessing the effects of various β-apocarotenoids on RXRα activation, β-apo-13-carotenone was identified as the most potent antagonist among tested compounds. The study utilized transactivation assays to measure the inhibition of 9cRA-induced gene expression, demonstrating significant antagonistic properties across multiple experiments .
Study 2: Tetramerization Induction
Another research effort focused on elucidating the mechanism behind β-apo-13-carotenone's effects on RXRα transcriptional activity. The findings revealed that this compound induces tetramerization of RXRα's LBD, which is critical for its function as a transcriptional regulator .
Study 3: Dietary Sources and Absorption
Research examining dietary sources indicated that β-apo-13-carotenone is present in various foods and can be absorbed into the bloodstream. However, its rapid degradation raises questions about its long-term bioactivity within the body .
Comparative Table of Biological Activities
Compound | Biological Activity | Effective Concentration |
---|---|---|
This compound | Antagonizes RXRα activation | 1 nM |
UVI3003 | Antagonist but does not induce tetramerization | N/A |
Beta-Apo-12’-Carotenoic Acid | Moderate antagonist | 96% inhibition |
Beta-Apo-8’-Carotenal | Partial antagonist | 73%-78% inhibition |
Q & A
Basic Research Questions
Q. What experimental methods are used to identify and characterize β-apo-13-carotenone in enzymatic cleavage studies?
- β-Apo-13-carotenone is identified via high-performance liquid chromatography (HPLC) with retention time matching, coupled with ultraviolet/visible (UV/Vis) spectral analysis (absorption maxima ~350–450 nm). Confirmation involves chemical derivatization (e.g., oxime formation) and mass spectrometry (MS) for molecular weight validation. Enzymatic origin is confirmed by comparing incubations with/without intestinal homogenates or inhibitors like disulfiram .
Q. How is β-apo-13-carotenone detected in natural sources, and what methodological considerations apply?
- In plant matrices (e.g., orange-fleshed melons), β-apo-13-carotenone is extracted using organic solvents (hexane/acetone), purified via column chromatography, and quantified using HPLC with diode-array detection. Method validation includes spike-recovery tests and comparison with synthetic standards. Sophisticated MS platforms (e.g., LC-MS/MS) enhance specificity in complex matrices .
Q. What are the optimal conditions for studying β-carotene cleavage to β-apo-13-carotenone in vitro?
- Incubation systems require intestinal mucosa homogenates (1.5 mg/mL protein), β-carotene dissolved in tetrahydrofuran, and incubation at 37°C for ≤60 minutes. Reaction linearity is confirmed by time-course and substrate concentration curves. Negative controls (e.g., heat-inactivated enzymes) are critical to distinguish enzymatic vs. non-enzymatic cleavage .
Q. How do researchers differentiate enzymatic vs. non-enzymatic cleavage products of β-carotene?
- Enzymatic cleavage is validated by: (i) Dose-dependent inhibition with disulfiram (a cytochrome P450 inhibitor). (ii) Linear product formation with increasing protein concentration. (iii) Absence of β-apo-13-carotenone in inhibitor-treated or homogenate-free controls .
Advanced Research Questions
Q. What molecular mechanisms underlie β-apo-13-carotenone's antagonism of retinoid X receptor-alpha (RXRα)?
- β-Apo-13-carotenone binds RXRα's ligand-binding domain (LBD), inducing tetramerization (via size-exclusion chromatography and X-ray crystallography), which silences transcriptional activity. Unlike synthetic antagonists (e.g., UVI3003), it does not block coactivator binding but alters receptor oligomerization .
Q. How can contradictions in β-apo-13-carotenone's RXRα modulation be resolved experimentally?
- Discrepancies arise from assay design:
- Transactivation assays (RXRE-Luc reporter in COS-7 cells) show dose-dependent antagonism.
- Coactivator recruitment assays (Gal4-DBD:RXRαLBD hybrid) reveal no inhibition of 9cRA-induced binding.
Resolve via structural analysis (e.g., cryo-EM) of tetramerized RXRα and comparative studies with mutant receptors .
Q. What cell-based models are appropriate for studying β-apo-13-carotenone's bioactivity?
- Use COS-7 cells transiently transfected with RXRα and RXRE-Luc reporter for transactivation assays. For physiological relevance, employ cancer cell lines (e.g., MCF-7) to assess gene regulation (e.g., caspase-9 expression) via qPCR. Normalize data to housekeeping genes and include antagonist controls (UVI3003) .
Q. How do in vitro findings on β-apo-13-carotenone translate to in vivo models?
- In vitro enzymatic cleavage kinetics (e.g., linearity up to 60 minutes) may not reflect in vivo bioavailability due to absorption barriers and metabolism. Use stable isotope-labeled β-carotene in animal models (ferrets, rats) with LC-MS/MS quantification of β-apo-13-carotenone in plasma/tissues .
Q. What structural analysis techniques clarify β-apo-13-carotenone's interaction with nuclear receptors?
- X-ray crystallography of RXRαLBD bound to β-apo-13-carotenone identifies binding pockets and conformational changes.
- Small-angle X-ray scattering (SAXS) monitors tetramer formation in solution.
- Molecular dynamics simulations predict stability of receptor-ligand complexes .
Q. How does β-apo-13-carotenone's metabolism differ from other apocarotenoids?
- Unlike β-apo-14'-carotenal (a direct vitamin A precursor), β-apo-13-carotenone undergoes reductive metabolism to alcohol derivatives in liver microsomes. Track metabolites using radiolabeled compounds and NADPH-dependent enzyme assays .
Properties
IUPAC Name |
(3E,5E,7E)-6-methyl-8-(2,6,6-trimethylcyclohexen-1-yl)octa-3,5,7-trien-2-one | |
---|---|---|
Source | PubChem | |
URL | https://pubchem.ncbi.nlm.nih.gov | |
Description | Data deposited in or computed by PubChem | |
InChI |
InChI=1S/C18H26O/c1-14(8-6-10-16(3)19)11-12-17-15(2)9-7-13-18(17,4)5/h6,8,10-12H,7,9,13H2,1-5H3/b10-6+,12-11+,14-8+ | |
Source | PubChem | |
URL | https://pubchem.ncbi.nlm.nih.gov | |
Description | Data deposited in or computed by PubChem | |
InChI Key |
UBTNVRPIHJRBCI-LUXGDSJYSA-N | |
Source | PubChem | |
URL | https://pubchem.ncbi.nlm.nih.gov | |
Description | Data deposited in or computed by PubChem | |
Canonical SMILES |
CC1=C(C(CCC1)(C)C)C=CC(=CC=CC(=O)C)C | |
Source | PubChem | |
URL | https://pubchem.ncbi.nlm.nih.gov | |
Description | Data deposited in or computed by PubChem | |
Isomeric SMILES |
CC1=C(C(CCC1)(C)C)/C=C/C(=C/C=C/C(=O)C)/C | |
Source | PubChem | |
URL | https://pubchem.ncbi.nlm.nih.gov | |
Description | Data deposited in or computed by PubChem | |
Molecular Formula |
C18H26O | |
Source | PubChem | |
URL | https://pubchem.ncbi.nlm.nih.gov | |
Description | Data deposited in or computed by PubChem | |
Molecular Weight |
258.4 g/mol | |
Source | PubChem | |
URL | https://pubchem.ncbi.nlm.nih.gov | |
Description | Data deposited in or computed by PubChem | |
Retrosynthesis Analysis
AI-Powered Synthesis Planning: Our tool employs the Template_relevance Pistachio, Template_relevance Bkms_metabolic, Template_relevance Pistachio_ringbreaker, Template_relevance Reaxys, Template_relevance Reaxys_biocatalysis model, leveraging a vast database of chemical reactions to predict feasible synthetic routes.
One-Step Synthesis Focus: Specifically designed for one-step synthesis, it provides concise and direct routes for your target compounds, streamlining the synthesis process.
Accurate Predictions: Utilizing the extensive PISTACHIO, BKMS_METABOLIC, PISTACHIO_RINGBREAKER, REAXYS, REAXYS_BIOCATALYSIS database, our tool offers high-accuracy predictions, reflecting the latest in chemical research and data.
Strategy Settings
Precursor scoring | Relevance Heuristic |
---|---|
Min. plausibility | 0.01 |
Model | Template_relevance |
Template Set | Pistachio/Bkms_metabolic/Pistachio_ringbreaker/Reaxys/Reaxys_biocatalysis |
Top-N result to add to graph | 6 |
Feasible Synthetic Routes
Disclaimer and Information on In-Vitro Research Products
Please be aware that all articles and product information presented on BenchChem are intended solely for informational purposes. The products available for purchase on BenchChem are specifically designed for in-vitro studies, which are conducted outside of living organisms. In-vitro studies, derived from the Latin term "in glass," involve experiments performed in controlled laboratory settings using cells or tissues. It is important to note that these products are not categorized as medicines or drugs, and they have not received approval from the FDA for the prevention, treatment, or cure of any medical condition, ailment, or disease. We must emphasize that any form of bodily introduction of these products into humans or animals is strictly prohibited by law. It is essential to adhere to these guidelines to ensure compliance with legal and ethical standards in research and experimentation.