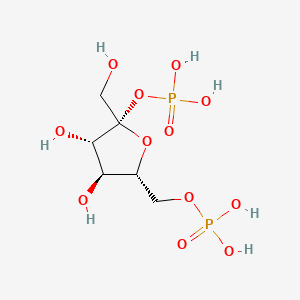
Fructose 2,6-biphosphate
Overview
Description
Fructose 2,6-biphosphate is a metabolite that plays a crucial role in the regulation of glycolysis and gluconeogenesis. It is known for its ability to allosterically affect the activity of key enzymes such as phosphofructokinase 1 and fructose 1,6-bisphosphatase . This compound is synthesized and broken down by the bifunctional enzyme phosphofructokinase 2/fructose 2,6-bisphosphatase .
Preparation Methods
Synthetic Routes and Reaction Conditions: Fructose 2,6-biphosphate is synthesized in the cytosol through the phosphorylation of fructose 6-phosphate by the enzyme phosphofructokinase 2 using ATP . The reaction conditions typically involve a dephosphorylated state of the enzyme, which is favored by high levels of insulin .
Industrial Production Methods: This enzyme can then be used to catalyze the production of this compound from fructose 6-phosphate and ATP under controlled conditions .
Chemical Reactions Analysis
Types of Reactions: Fructose 2,6-biphosphate primarily undergoes phosphorylation and dephosphorylation reactions. It is phosphorylated by phosphofructokinase 2 and dephosphorylated by fructose 2,6-bisphosphatase .
Common Reagents and Conditions:
Phosphorylation: ATP is used as the phosphate donor in the presence of phosphofructokinase 2.
Dephosphorylation: The reaction is catalyzed by fructose 2,6-bisphosphatase, which removes the phosphate group.
Major Products:
Phosphorylation: this compound is formed from fructose 6-phosphate and ATP.
Dephosphorylation: Fructose 6-phosphate and inorganic phosphate are the products of the dephosphorylation reaction.
Scientific Research Applications
Fructose 2,6-biphosphate has a wide range of applications in scientific research:
Mechanism of Action
Fructose 2,6-biphosphate exerts its effects by allosterically modulating the activity of key enzymes involved in glycolysis and gluconeogenesis. It activates phosphofructokinase 1 by increasing its affinity for fructose 6-phosphate and decreasing its affinity for inhibitory ATP and citrate . Conversely, it inhibits fructose 1,6-bisphosphatase, thereby regulating the balance between glycolysis and gluconeogenesis .
Comparison with Similar Compounds
Fructose 1,6-bisphosphate: Another important regulator of glycolysis, but it is involved in different steps of the pathway.
Glucose 6-phosphate: Plays a role in glycolysis and gluconeogenesis but does not have the same regulatory effects as fructose 2,6-biphosphate.
Uniqueness: this compound is unique in its ability to allosterically regulate both glycolysis and gluconeogenesis by modulating the activity of phosphofructokinase 1 and fructose 1,6-bisphosphatase . This dual regulatory role makes it a critical molecule in maintaining metabolic balance.
Properties
IUPAC Name |
[(2S,3S,4S,5R)-3,4-dihydroxy-2-(hydroxymethyl)-5-(phosphonooxymethyl)oxolan-2-yl] dihydrogen phosphate | |
---|---|---|
Source | PubChem | |
URL | https://pubchem.ncbi.nlm.nih.gov | |
Description | Data deposited in or computed by PubChem | |
InChI |
InChI=1S/C6H14O12P2/c7-2-6(18-20(13,14)15)5(9)4(8)3(17-6)1-16-19(10,11)12/h3-5,7-9H,1-2H2,(H2,10,11,12)(H2,13,14,15)/t3-,4-,5+,6+/m1/s1 | |
Source | PubChem | |
URL | https://pubchem.ncbi.nlm.nih.gov | |
Description | Data deposited in or computed by PubChem | |
InChI Key |
YXWOAJXNVLXPMU-ZXXMMSQZSA-N | |
Source | PubChem | |
URL | https://pubchem.ncbi.nlm.nih.gov | |
Description | Data deposited in or computed by PubChem | |
Canonical SMILES |
C(C1C(C(C(O1)(CO)OP(=O)(O)O)O)O)OP(=O)(O)O | |
Source | PubChem | |
URL | https://pubchem.ncbi.nlm.nih.gov | |
Description | Data deposited in or computed by PubChem | |
Isomeric SMILES |
C([C@@H]1[C@H]([C@@H]([C@](O1)(CO)OP(=O)(O)O)O)O)OP(=O)(O)O | |
Source | PubChem | |
URL | https://pubchem.ncbi.nlm.nih.gov | |
Description | Data deposited in or computed by PubChem | |
Molecular Formula |
C6H14O12P2 | |
Source | PubChem | |
URL | https://pubchem.ncbi.nlm.nih.gov | |
Description | Data deposited in or computed by PubChem | |
DSSTOX Substance ID |
DTXSID90897603 | |
Record name | Fructose 2,6-diphosphate | |
Source | EPA DSSTox | |
URL | https://comptox.epa.gov/dashboard/DTXSID90897603 | |
Description | DSSTox provides a high quality public chemistry resource for supporting improved predictive toxicology. | |
Molecular Weight |
340.12 g/mol | |
Source | PubChem | |
URL | https://pubchem.ncbi.nlm.nih.gov | |
Description | Data deposited in or computed by PubChem | |
Physical Description |
Solid | |
Record name | D-Fructose 2,6-bisphosphate | |
Source | Human Metabolome Database (HMDB) | |
URL | http://www.hmdb.ca/metabolites/HMDB0001047 | |
Description | The Human Metabolome Database (HMDB) is a freely available electronic database containing detailed information about small molecule metabolites found in the human body. | |
Explanation | HMDB is offered to the public as a freely available resource. Use and re-distribution of the data, in whole or in part, for commercial purposes requires explicit permission of the authors and explicit acknowledgment of the source material (HMDB) and the original publication (see the HMDB citing page). We ask that users who download significant portions of the database cite the HMDB paper in any resulting publications. | |
CAS No. |
79082-92-1 | |
Record name | Fructose 2,6-diphosphate | |
Source | CAS Common Chemistry | |
URL | https://commonchemistry.cas.org/detail?cas_rn=79082-92-1 | |
Description | CAS Common Chemistry is an open community resource for accessing chemical information. Nearly 500,000 chemical substances from CAS REGISTRY cover areas of community interest, including common and frequently regulated chemicals, and those relevant to high school and undergraduate chemistry classes. This chemical information, curated by our expert scientists, is provided in alignment with our mission as a division of the American Chemical Society. | |
Explanation | The data from CAS Common Chemistry is provided under a CC-BY-NC 4.0 license, unless otherwise stated. | |
Record name | Fructose 2,6-diphosphate | |
Source | ChemIDplus | |
URL | https://pubchem.ncbi.nlm.nih.gov/substance/?source=chemidplus&sourceid=0079082921 | |
Description | ChemIDplus is a free, web search system that provides access to the structure and nomenclature authority files used for the identification of chemical substances cited in National Library of Medicine (NLM) databases, including the TOXNET system. | |
Record name | Fructose 2,6-diphosphate | |
Source | EPA DSSTox | |
URL | https://comptox.epa.gov/dashboard/DTXSID90897603 | |
Description | DSSTox provides a high quality public chemistry resource for supporting improved predictive toxicology. | |
Record name | Fructose 2,6-diphosphate | |
Source | FDA Global Substance Registration System (GSRS) | |
URL | https://gsrs.ncats.nih.gov/ginas/app/beta/substances/SE96VBE4LU | |
Description | The FDA Global Substance Registration System (GSRS) enables the efficient and accurate exchange of information on what substances are in regulated products. Instead of relying on names, which vary across regulatory domains, countries, and regions, the GSRS knowledge base makes it possible for substances to be defined by standardized, scientific descriptions. | |
Explanation | Unless otherwise noted, the contents of the FDA website (www.fda.gov), both text and graphics, are not copyrighted. They are in the public domain and may be republished, reprinted and otherwise used freely by anyone without the need to obtain permission from FDA. Credit to the U.S. Food and Drug Administration as the source is appreciated but not required. | |
Record name | D-Fructose 2,6-bisphosphate | |
Source | Human Metabolome Database (HMDB) | |
URL | http://www.hmdb.ca/metabolites/HMDB0001047 | |
Description | The Human Metabolome Database (HMDB) is a freely available electronic database containing detailed information about small molecule metabolites found in the human body. | |
Explanation | HMDB is offered to the public as a freely available resource. Use and re-distribution of the data, in whole or in part, for commercial purposes requires explicit permission of the authors and explicit acknowledgment of the source material (HMDB) and the original publication (see the HMDB citing page). We ask that users who download significant portions of the database cite the HMDB paper in any resulting publications. | |
Retrosynthesis Analysis
AI-Powered Synthesis Planning: Our tool employs the Template_relevance Pistachio, Template_relevance Bkms_metabolic, Template_relevance Pistachio_ringbreaker, Template_relevance Reaxys, Template_relevance Reaxys_biocatalysis model, leveraging a vast database of chemical reactions to predict feasible synthetic routes.
One-Step Synthesis Focus: Specifically designed for one-step synthesis, it provides concise and direct routes for your target compounds, streamlining the synthesis process.
Accurate Predictions: Utilizing the extensive PISTACHIO, BKMS_METABOLIC, PISTACHIO_RINGBREAKER, REAXYS, REAXYS_BIOCATALYSIS database, our tool offers high-accuracy predictions, reflecting the latest in chemical research and data.
Strategy Settings
Precursor scoring | Relevance Heuristic |
---|---|
Min. plausibility | 0.01 |
Model | Template_relevance |
Template Set | Pistachio/Bkms_metabolic/Pistachio_ringbreaker/Reaxys/Reaxys_biocatalysis |
Top-N result to add to graph | 6 |
Feasible Synthetic Routes
Disclaimer and Information on In-Vitro Research Products
Please be aware that all articles and product information presented on BenchChem are intended solely for informational purposes. The products available for purchase on BenchChem are specifically designed for in-vitro studies, which are conducted outside of living organisms. In-vitro studies, derived from the Latin term "in glass," involve experiments performed in controlled laboratory settings using cells or tissues. It is important to note that these products are not categorized as medicines or drugs, and they have not received approval from the FDA for the prevention, treatment, or cure of any medical condition, ailment, or disease. We must emphasize that any form of bodily introduction of these products into humans or animals is strictly prohibited by law. It is essential to adhere to these guidelines to ensure compliance with legal and ethical standards in research and experimentation.