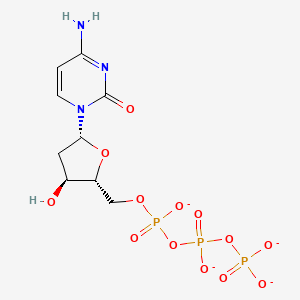
2'-Deoxycytidine-5'-triphosphate
Overview
Description
Deoxycytidine triphosphate is a nucleoside triphosphate that contains the pyrimidine base cytosine. It plays a crucial role in DNA synthesis, serving as a substrate for DNA polymerase enzymes. The triphosphate group in deoxycytidine triphosphate contains high-energy phosphoanhydride bonds, which release energy upon hydrolysis, facilitating the incorporation of deoxycytidine into a newly synthesized DNA strand .
Mechanism of Action
Target of Action
2’-Deoxycytidine-5’-triphosphate (dCTP) primarily targets several enzymes, including Anaerobic ribonucleoside-triphosphate reductase , Deoxycytidine triphosphate deaminase , DNA polymerase I, thermostable , and Ribonucleoside-diphosphate reductase 2 subunit alpha . These enzymes play crucial roles in DNA synthesis and regulation, making them key targets for dCTP.
Mode of Action
dCTP interacts with its targets by serving as a substrate for DNA synthesis. It is used by DNA polymerases and reverse transcriptases to synthesize DNA . Additionally, dCTP acts as an allosteric regulator of deoxycytidylate (dCMP) deaminase , influencing the enzyme’s activity and thereby affecting DNA synthesis.
Biochemical Pathways
dCTP is synthesized either by the de novo pathway or from the multiple phosphorylation steps of cytidine . It is then deaminated to deoxyuridine monophosphate (dUMP), which is convertible to deoxythymidine triphosphate (dTTP) . This process is part of the nucleotide metabolism pathway, which is essential for DNA synthesis and repair.
Pharmacokinetics
It is known that the compound is utilized by cells for dna polymerase-catalyzed synthesis of dna .
Result of Action
The primary result of dCTP’s action is the synthesis of DNA. By serving as a substrate for DNA polymerases and reverse transcriptases, dCTP contributes to the creation of new DNA strands . This is a crucial process for cell replication and function.
Action Environment
The action of dCTP is influenced by various environmental factors within the cell. For instance, the presence of other nucleotides and enzymes can affect the efficiency of dCTP incorporation into DNA. Additionally, the pH and ion concentrations in the cellular environment can impact the stability and efficacy of dCTP .
Biochemical Analysis
Biochemical Properties
2’-dCTP plays a significant role in biochemical reactions. It is synthesized either by the de novo pathway or from the multiple phosphorylation steps of cytidine . It interacts with various enzymes, proteins, and other biomolecules. For instance, it is used by DNA polymerases or reverse transcriptases to synthesize DNA . It also acts as an allosteric regulator of deoxycytidylate (dCMP) deaminase .
Cellular Effects
The effects of 2’-dCTP on various types of cells and cellular processes are profound. It influences cell function by being incorporated into the DNA during replication . This incorporation can affect cell signaling pathways, gene expression, and cellular metabolism. For example, it can lead to changes in gene expression by altering the DNA sequence.
Molecular Mechanism
The molecular mechanism of 2’-dCTP involves its incorporation into a newly synthesized strand of DNA by DNA polymerase enzymes . This process is driven by the energy liberated when the high-energy phosphoanhydride bonds in the triphosphate group of 2’-dCTP are hydrolyzed . The incorporation of 2’-dCTP into DNA can lead to changes in gene expression and can influence the activity of various enzymes and proteins.
Metabolic Pathways
2’-dCTP is involved in several metabolic pathways. It is synthesized either by the de novo pathway or from the multiple phosphorylation steps of cytidine . After its synthesis, 2’-dCTP can be deaminated to deoxyuridine monophosphate (dUMP), which can be converted to deoxythymidine triphosphate (dTTP) .
Preparation Methods
Synthetic Routes and Reaction Conditions: Deoxycytidine triphosphate can be synthesized enzymatically or chemically. Enzymatic synthesis involves the use of recombinant Escherichia coli displaying nucleotide kinases on the cell surface. These bacteria catalyze the conversion of cytidine-5′-triphosphate to deoxycytidine-5′-triphosphate with high efficiency . Chemical synthesis typically involves the phosphorylation of nucleosides using phosphorus oxychloride in organic solvents like pyridine or dimethylformamide .
Industrial Production Methods: Industrial production of deoxycytidine triphosphate often employs enzymatic methods due to their higher efficiency and lower environmental impact compared to chemical methods. The process involves two sequential enzymatic phosphorylation reactions starting from deoxynucleoside monophosphates to deoxynucleoside diphosphates and finally to deoxynucleoside triphosphates .
Chemical Reactions Analysis
Types of Reactions: Deoxycytidine triphosphate undergoes various chemical reactions, including:
Common Reagents and Conditions:
Hydrolysis: Typically occurs in aqueous solutions at physiological pH and temperature.
Deamination: Catalyzed by deoxycytidine triphosphate deaminase in the presence of water.
Major Products:
Hydrolysis: Produces deoxycytidine monophosphate and pyrophosphate.
Deamination: Produces deoxyuridine triphosphate.
Scientific Research Applications
Deoxycytidine triphosphate is widely used in various scientific research fields:
Comparison with Similar Compounds
- Deoxyadenosine triphosphate
- Deoxyguanosine triphosphate
- Deoxythymidine triphosphate
Comparison: Deoxycytidine triphosphate is unique among nucleoside triphosphates due to its pyrimidine base cytosine. While deoxyadenosine triphosphate, deoxyguanosine triphosphate, and deoxythymidine triphosphate contain purine or pyrimidine bases, deoxycytidine triphosphate specifically pairs with guanine during DNA synthesis, ensuring the fidelity of the genetic code .
Properties
IUPAC Name |
[[(2R,3S,5R)-5-(4-amino-2-oxopyrimidin-1-yl)-3-hydroxyoxolan-2-yl]methoxy-hydroxyphosphoryl] phosphono hydrogen phosphate | |
---|---|---|
Source | PubChem | |
URL | https://pubchem.ncbi.nlm.nih.gov | |
Description | Data deposited in or computed by PubChem | |
InChI |
InChI=1S/C9H16N3O13P3/c10-7-1-2-12(9(14)11-7)8-3-5(13)6(23-8)4-22-27(18,19)25-28(20,21)24-26(15,16)17/h1-2,5-6,8,13H,3-4H2,(H,18,19)(H,20,21)(H2,10,11,14)(H2,15,16,17)/t5-,6+,8+/m0/s1 | |
Source | PubChem | |
URL | https://pubchem.ncbi.nlm.nih.gov | |
Description | Data deposited in or computed by PubChem | |
InChI Key |
RGWHQCVHVJXOKC-SHYZEUOFSA-N | |
Source | PubChem | |
URL | https://pubchem.ncbi.nlm.nih.gov | |
Description | Data deposited in or computed by PubChem | |
Canonical SMILES |
C1C(C(OC1N2C=CC(=NC2=O)N)COP(=O)(O)OP(=O)(O)OP(=O)(O)O)O | |
Source | PubChem | |
URL | https://pubchem.ncbi.nlm.nih.gov | |
Description | Data deposited in or computed by PubChem | |
Isomeric SMILES |
C1[C@@H]([C@H](O[C@H]1N2C=CC(=NC2=O)N)COP(=O)(O)OP(=O)(O)OP(=O)(O)O)O | |
Source | PubChem | |
URL | https://pubchem.ncbi.nlm.nih.gov | |
Description | Data deposited in or computed by PubChem | |
Molecular Formula |
C9H16N3O13P3 | |
Source | PubChem | |
URL | https://pubchem.ncbi.nlm.nih.gov | |
Description | Data deposited in or computed by PubChem | |
DSSTOX Substance ID |
DTXSID60942755 | |
Record name | 2′-Deoxycytidine 5′-(tetrahydrogen triphosphate) | |
Source | EPA DSSTox | |
URL | https://comptox.epa.gov/dashboard/DTXSID60942755 | |
Description | DSSTox provides a high quality public chemistry resource for supporting improved predictive toxicology. | |
Molecular Weight |
467.16 g/mol | |
Source | PubChem | |
URL | https://pubchem.ncbi.nlm.nih.gov | |
Description | Data deposited in or computed by PubChem | |
Physical Description |
Solid | |
Record name | dCTP | |
Source | Human Metabolome Database (HMDB) | |
URL | http://www.hmdb.ca/metabolites/HMDB0000998 | |
Description | The Human Metabolome Database (HMDB) is a freely available electronic database containing detailed information about small molecule metabolites found in the human body. | |
Explanation | HMDB is offered to the public as a freely available resource. Use and re-distribution of the data, in whole or in part, for commercial purposes requires explicit permission of the authors and explicit acknowledgment of the source material (HMDB) and the original publication (see the HMDB citing page). We ask that users who download significant portions of the database cite the HMDB paper in any resulting publications. | |
CAS No. |
2056-98-6 | |
Record name | dCTP | |
Source | CAS Common Chemistry | |
URL | https://commonchemistry.cas.org/detail?cas_rn=2056-98-6 | |
Description | CAS Common Chemistry is an open community resource for accessing chemical information. Nearly 500,000 chemical substances from CAS REGISTRY cover areas of community interest, including common and frequently regulated chemicals, and those relevant to high school and undergraduate chemistry classes. This chemical information, curated by our expert scientists, is provided in alignment with our mission as a division of the American Chemical Society. | |
Explanation | The data from CAS Common Chemistry is provided under a CC-BY-NC 4.0 license, unless otherwise stated. | |
Record name | 2'-Deoxycytidine 5'-triphosphate | |
Source | ChemIDplus | |
URL | https://pubchem.ncbi.nlm.nih.gov/substance/?source=chemidplus&sourceid=0002056986 | |
Description | ChemIDplus is a free, web search system that provides access to the structure and nomenclature authority files used for the identification of chemical substances cited in National Library of Medicine (NLM) databases, including the TOXNET system. | |
Record name | 2'-Deoxycytidine 5'-triphosphate | |
Source | DrugBank | |
URL | https://www.drugbank.ca/drugs/DB03258 | |
Description | The DrugBank database is a unique bioinformatics and cheminformatics resource that combines detailed drug (i.e. chemical, pharmacological and pharmaceutical) data with comprehensive drug target (i.e. sequence, structure, and pathway) information. | |
Explanation | Creative Common's Attribution-NonCommercial 4.0 International License (http://creativecommons.org/licenses/by-nc/4.0/legalcode) | |
Record name | 2′-Deoxycytidine 5′-(tetrahydrogen triphosphate) | |
Source | EPA DSSTox | |
URL | https://comptox.epa.gov/dashboard/DTXSID60942755 | |
Description | DSSTox provides a high quality public chemistry resource for supporting improved predictive toxicology. | |
Record name | Cytidine 5'-(tetrahydrogen triphosphate), 2'-deoxy- | |
Source | European Chemicals Agency (ECHA) | |
URL | https://echa.europa.eu/substance-information/-/substanceinfo/100.016.504 | |
Description | The European Chemicals Agency (ECHA) is an agency of the European Union which is the driving force among regulatory authorities in implementing the EU's groundbreaking chemicals legislation for the benefit of human health and the environment as well as for innovation and competitiveness. | |
Explanation | Use of the information, documents and data from the ECHA website is subject to the terms and conditions of this Legal Notice, and subject to other binding limitations provided for under applicable law, the information, documents and data made available on the ECHA website may be reproduced, distributed and/or used, totally or in part, for non-commercial purposes provided that ECHA is acknowledged as the source: "Source: European Chemicals Agency, http://echa.europa.eu/". Such acknowledgement must be included in each copy of the material. ECHA permits and encourages organisations and individuals to create links to the ECHA website under the following cumulative conditions: Links can only be made to webpages that provide a link to the Legal Notice page. | |
Record name | dCTP | |
Source | Human Metabolome Database (HMDB) | |
URL | http://www.hmdb.ca/metabolites/HMDB0000998 | |
Description | The Human Metabolome Database (HMDB) is a freely available electronic database containing detailed information about small molecule metabolites found in the human body. | |
Explanation | HMDB is offered to the public as a freely available resource. Use and re-distribution of the data, in whole or in part, for commercial purposes requires explicit permission of the authors and explicit acknowledgment of the source material (HMDB) and the original publication (see the HMDB citing page). We ask that users who download significant portions of the database cite the HMDB paper in any resulting publications. | |
Retrosynthesis Analysis
AI-Powered Synthesis Planning: Our tool employs the Template_relevance Pistachio, Template_relevance Bkms_metabolic, Template_relevance Pistachio_ringbreaker, Template_relevance Reaxys, Template_relevance Reaxys_biocatalysis model, leveraging a vast database of chemical reactions to predict feasible synthetic routes.
One-Step Synthesis Focus: Specifically designed for one-step synthesis, it provides concise and direct routes for your target compounds, streamlining the synthesis process.
Accurate Predictions: Utilizing the extensive PISTACHIO, BKMS_METABOLIC, PISTACHIO_RINGBREAKER, REAXYS, REAXYS_BIOCATALYSIS database, our tool offers high-accuracy predictions, reflecting the latest in chemical research and data.
Strategy Settings
Precursor scoring | Relevance Heuristic |
---|---|
Min. plausibility | 0.01 |
Model | Template_relevance |
Template Set | Pistachio/Bkms_metabolic/Pistachio_ringbreaker/Reaxys/Reaxys_biocatalysis |
Top-N result to add to graph | 6 |
Feasible Synthetic Routes
Disclaimer and Information on In-Vitro Research Products
Please be aware that all articles and product information presented on BenchChem are intended solely for informational purposes. The products available for purchase on BenchChem are specifically designed for in-vitro studies, which are conducted outside of living organisms. In-vitro studies, derived from the Latin term "in glass," involve experiments performed in controlled laboratory settings using cells or tissues. It is important to note that these products are not categorized as medicines or drugs, and they have not received approval from the FDA for the prevention, treatment, or cure of any medical condition, ailment, or disease. We must emphasize that any form of bodily introduction of these products into humans or animals is strictly prohibited by law. It is essential to adhere to these guidelines to ensure compliance with legal and ethical standards in research and experimentation.