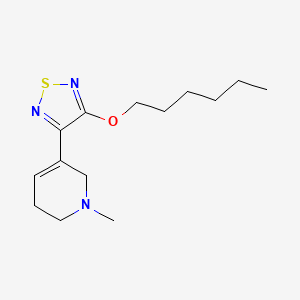
Xanomeline
Overview
Description
Xanomeline is a small molecule muscarinic acetylcholine receptor agonist. It was first synthesized in a collaboration between Eli Lilly and Novo Nordisk as an investigational therapeutic for central nervous system disorders . This compound primarily targets the muscarinic M1 and M4 receptor subtypes, which are involved in regulating key neurotransmitter circuits in the brain .
Scientific Research Applications
Xanomeline has been extensively studied for its potential therapeutic applications, particularly in the treatment of central nervous system disorders such as schizophrenia and Alzheimer’s disease . It has shown promise in regulating key neurotransmitter circuits, including acetylcholine, dopamine, and glutamate, which are disrupted in these disorders .
In addition to its use in medicine, this compound is also used in research to study the muscarinic acetylcholine receptor family and their role in various physiological processes . Its ability to selectively target M1 and M4 receptors makes it a valuable tool for understanding the function of these receptors in the brain.
Mechanism of Action
Xanomeline exerts its effects by acting as an agonist at muscarinic acetylcholine receptors, specifically the M1 and M4 subtypes . By stimulating these receptors, this compound helps to rebalance neurotransmitter circuits that are disrupted in conditions like schizophrenia and Alzheimer’s disease . This rebalancing involves modulation of acetylcholine, dopamine, and glutamate pathways, leading to improvements in cognitive and behavioral symptoms .
Future Directions
Biochemical Analysis
Biochemical Properties
Xanomeline plays a significant role in biochemical reactions by acting as an agonist for the muscarinic acetylcholine receptor family, which includes five subtypes: M1, M2, M3, M4, and M5 . It binds with near identical affinity to all five subtypes but exhibits functional selectivity for the M1 and M4 receptors . The interaction of this compound with these receptors leads to the modulation of neurotransmitter circuits, including acetylcholine, dopamine, and glutamate . This modulation is crucial for rebalancing neurotransmitter circuits disrupted in neuropsychiatric and neurological diseases .
Cellular Effects
This compound influences various cellular processes by targeting muscarinic receptors, particularly M1 and M4 . In the central nervous system, this compound regulates key dopaminergic and glutamatergic circuits, which are essential for cognitive function and behavior . It has been shown to protect cortical cells from oxygen-glucose deprivation and increase the release of soluble amyloid precursor protein from cells . Additionally, this compound’s agonistic activity at the M1 receptor impacts cell signaling pathways, gene expression, and cellular metabolism .
Molecular Mechanism
At the molecular level, this compound exerts its effects through the stimulation of muscarinic M1 and M4 receptors . This stimulation leads to the rebalancing of key neurotransmitter circuits, including acetylcholine, dopamine, and glutamate . This compound’s binding interactions with these receptors result in the activation of downstream signaling pathways that regulate neurotransmitter release and neural circuit function . The compound’s mechanism of action is hypothesized to involve the modulation of neurotransmitter circuits disrupted in schizophrenia and related diseases .
Temporal Effects in Laboratory Settings
In laboratory settings, the effects of this compound have been observed to change over time. This compound is rapidly absorbed and extensively biotransformed into metabolites . The compound exhibits a rapid initial elimination phase followed by a slower terminal elimination phase . Long-term studies have shown that this compound can lead to sustained increases in acetylcholine levels and modulation of dopaminergic and glutamatergic circuits . Its development was initially discontinued due to cholinergic side effects, which were later mitigated through the co-formulation with trospium .
Dosage Effects in Animal Models
In animal models, the effects of this compound vary with different dosages. Studies have shown that this compound can reverse amphetamine- and scopolamine-induced disruptions in latent inhibition, which is predictive of its activity against positive symptoms of schizophrenia . The compound also alleviates MK801-induced abnormalities, suggesting efficacy against negative and cognitive symptoms . High doses of this compound can lead to adverse effects such as dystonia in animal models .
Metabolic Pathways
This compound is involved in metabolic pathways that include its biotransformation into various metabolites . The compound is rapidly absorbed and extensively metabolized, with a significant portion of the dose recovered in urine and feces . The metabolic pathways of this compound involve interactions with enzymes that facilitate its biotransformation and elimination from the body .
Transport and Distribution
This compound is rapidly and extensively absorbed after oral administration . It has a high brain-to-plasma ratio, favoring its entry into the brain . The compound’s distribution within cells and tissues is influenced by its lipophilicity and absence of hydrogen bond donors . This compound’s transport and distribution are also affected by its interactions with transporters and binding proteins that facilitate its localization and accumulation in specific tissues .
Subcellular Localization
This compound’s subcellular localization is primarily within the central nervous system, where it targets muscarinic receptors . The compound’s activity and function are influenced by its localization to specific compartments and organelles within cells . This compound’s targeting signals and post-translational modifications direct it to areas where it can effectively modulate neurotransmitter circuits and cellular processes .
Preparation Methods
The synthesis of xanomeline involves several steps. The key intermediate is 3-(4-Hexoxy-1,2,5-thiadiazol-3-yl)-1-methyl-5,6-dihydro-2H-pyridine . The synthetic route typically includes the following steps:
Formation of the thiadiazole ring: This involves the reaction of appropriate starting materials under specific conditions to form the thiadiazole ring.
Attachment of the hexoxy group: The hexoxy group is introduced through a substitution reaction.
Formation of the pyridine ring: The final step involves the formation of the pyridine ring, completing the synthesis of this compound.
Industrial production methods for this compound are not widely documented, but they likely involve optimization of the above synthetic route to ensure high yield and purity.
Chemical Reactions Analysis
Xanomeline undergoes various chemical reactions, including:
Oxidation: this compound can be oxidized under specific conditions, leading to the formation of oxidized derivatives.
Reduction: Reduction reactions can modify the functional groups on this compound, potentially altering its pharmacological properties.
Substitution: This compound can undergo substitution reactions, where one functional group is replaced by another.
Common reagents used in these reactions include oxidizing agents like potassium permanganate, reducing agents like lithium aluminum hydride, and various nucleophiles for substitution reactions. The major products formed depend on the specific reaction conditions and reagents used.
Comparison with Similar Compounds
Xanomeline is unique in its selective targeting of M1 and M4 muscarinic receptors. Similar compounds include:
Acetylcholine: The natural neurotransmitter that this compound mimics in its action on muscarinic receptors.
Pilocarpine: Another muscarinic receptor agonist used in the treatment of glaucoma and dry mouth.
Compared to these compounds, this compound has a higher selectivity for M1 and M4 receptors, making it particularly useful for targeting central nervous system disorders .
properties
IUPAC Name |
3-hexoxy-4-(1-methyl-3,6-dihydro-2H-pyridin-5-yl)-1,2,5-thiadiazole | |
---|---|---|
Source | PubChem | |
URL | https://pubchem.ncbi.nlm.nih.gov | |
Description | Data deposited in or computed by PubChem | |
InChI |
InChI=1S/C14H23N3OS/c1-3-4-5-6-10-18-14-13(15-19-16-14)12-8-7-9-17(2)11-12/h8H,3-7,9-11H2,1-2H3 | |
Source | PubChem | |
URL | https://pubchem.ncbi.nlm.nih.gov | |
Description | Data deposited in or computed by PubChem | |
InChI Key |
JOLJIIDDOBNFHW-UHFFFAOYSA-N | |
Source | PubChem | |
URL | https://pubchem.ncbi.nlm.nih.gov | |
Description | Data deposited in or computed by PubChem | |
Canonical SMILES |
CCCCCCOC1=NSN=C1C2=CCCN(C2)C | |
Source | PubChem | |
URL | https://pubchem.ncbi.nlm.nih.gov | |
Description | Data deposited in or computed by PubChem | |
Molecular Formula |
C14H23N3OS | |
Source | PubChem | |
URL | https://pubchem.ncbi.nlm.nih.gov | |
Description | Data deposited in or computed by PubChem | |
DSSTOX Substance ID |
DTXSID60157286 | |
Record name | Xanomeline | |
Source | EPA DSSTox | |
URL | https://comptox.epa.gov/dashboard/DTXSID60157286 | |
Description | DSSTox provides a high quality public chemistry resource for supporting improved predictive toxicology. | |
Molecular Weight |
281.42 g/mol | |
Source | PubChem | |
URL | https://pubchem.ncbi.nlm.nih.gov | |
Description | Data deposited in or computed by PubChem | |
CAS RN |
131986-45-3 | |
Record name | Xanomeline | |
Source | CAS Common Chemistry | |
URL | https://commonchemistry.cas.org/detail?cas_rn=131986-45-3 | |
Description | CAS Common Chemistry is an open community resource for accessing chemical information. Nearly 500,000 chemical substances from CAS REGISTRY cover areas of community interest, including common and frequently regulated chemicals, and those relevant to high school and undergraduate chemistry classes. This chemical information, curated by our expert scientists, is provided in alignment with our mission as a division of the American Chemical Society. | |
Explanation | The data from CAS Common Chemistry is provided under a CC-BY-NC 4.0 license, unless otherwise stated. | |
Record name | Xanomeline [USAN:INN] | |
Source | ChemIDplus | |
URL | https://pubchem.ncbi.nlm.nih.gov/substance/?source=chemidplus&sourceid=0131986453 | |
Description | ChemIDplus is a free, web search system that provides access to the structure and nomenclature authority files used for the identification of chemical substances cited in National Library of Medicine (NLM) databases, including the TOXNET system. | |
Record name | Xanomeline | |
Source | DrugBank | |
URL | https://www.drugbank.ca/drugs/DB15357 | |
Description | The DrugBank database is a unique bioinformatics and cheminformatics resource that combines detailed drug (i.e. chemical, pharmacological and pharmaceutical) data with comprehensive drug target (i.e. sequence, structure, and pathway) information. | |
Explanation | Creative Common's Attribution-NonCommercial 4.0 International License (http://creativecommons.org/licenses/by-nc/4.0/legalcode) | |
Record name | Xanomeline | |
Source | EPA DSSTox | |
URL | https://comptox.epa.gov/dashboard/DTXSID60157286 | |
Description | DSSTox provides a high quality public chemistry resource for supporting improved predictive toxicology. | |
Record name | Xanomeline | |
Source | European Chemicals Agency (ECHA) | |
URL | https://echa.europa.eu/information-on-chemicals | |
Description | The European Chemicals Agency (ECHA) is an agency of the European Union which is the driving force among regulatory authorities in implementing the EU's groundbreaking chemicals legislation for the benefit of human health and the environment as well as for innovation and competitiveness. | |
Explanation | Use of the information, documents and data from the ECHA website is subject to the terms and conditions of this Legal Notice, and subject to other binding limitations provided for under applicable law, the information, documents and data made available on the ECHA website may be reproduced, distributed and/or used, totally or in part, for non-commercial purposes provided that ECHA is acknowledged as the source: "Source: European Chemicals Agency, http://echa.europa.eu/". Such acknowledgement must be included in each copy of the material. ECHA permits and encourages organisations and individuals to create links to the ECHA website under the following cumulative conditions: Links can only be made to webpages that provide a link to the Legal Notice page. | |
Record name | XANOMELINE | |
Source | FDA Global Substance Registration System (GSRS) | |
URL | https://gsrs.ncats.nih.gov/ginas/app/beta/substances/9ORI6L73CJ | |
Description | The FDA Global Substance Registration System (GSRS) enables the efficient and accurate exchange of information on what substances are in regulated products. Instead of relying on names, which vary across regulatory domains, countries, and regions, the GSRS knowledge base makes it possible for substances to be defined by standardized, scientific descriptions. | |
Explanation | Unless otherwise noted, the contents of the FDA website (www.fda.gov), both text and graphics, are not copyrighted. They are in the public domain and may be republished, reprinted and otherwise used freely by anyone without the need to obtain permission from FDA. Credit to the U.S. Food and Drug Administration as the source is appreciated but not required. | |
Retrosynthesis Analysis
AI-Powered Synthesis Planning: Our tool employs the Template_relevance Pistachio, Template_relevance Bkms_metabolic, Template_relevance Pistachio_ringbreaker, Template_relevance Reaxys, Template_relevance Reaxys_biocatalysis model, leveraging a vast database of chemical reactions to predict feasible synthetic routes.
One-Step Synthesis Focus: Specifically designed for one-step synthesis, it provides concise and direct routes for your target compounds, streamlining the synthesis process.
Accurate Predictions: Utilizing the extensive PISTACHIO, BKMS_METABOLIC, PISTACHIO_RINGBREAKER, REAXYS, REAXYS_BIOCATALYSIS database, our tool offers high-accuracy predictions, reflecting the latest in chemical research and data.
Strategy Settings
Precursor scoring | Relevance Heuristic |
---|---|
Min. plausibility | 0.01 |
Model | Template_relevance |
Template Set | Pistachio/Bkms_metabolic/Pistachio_ringbreaker/Reaxys/Reaxys_biocatalysis |
Top-N result to add to graph | 6 |
Feasible Synthetic Routes
Disclaimer and Information on In-Vitro Research Products
Please be aware that all articles and product information presented on BenchChem are intended solely for informational purposes. The products available for purchase on BenchChem are specifically designed for in-vitro studies, which are conducted outside of living organisms. In-vitro studies, derived from the Latin term "in glass," involve experiments performed in controlled laboratory settings using cells or tissues. It is important to note that these products are not categorized as medicines or drugs, and they have not received approval from the FDA for the prevention, treatment, or cure of any medical condition, ailment, or disease. We must emphasize that any form of bodily introduction of these products into humans or animals is strictly prohibited by law. It is essential to adhere to these guidelines to ensure compliance with legal and ethical standards in research and experimentation.