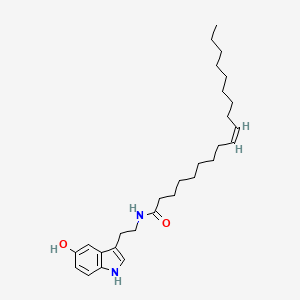
Oleoyl Serotonin
Overview
Description
Oleoyl Serotonin (OS) is a novel bioactive compound identified in the venom of Stephanoconus snails, characterized by its unique structure combining a serotonin moiety linked to an oleoyl fatty acid chain . OS functions as a competitive antagonist of cannabinoid receptors CB1 and CB2, with demonstrated efficacy in reversing cannabinoid receptor agonist-induced cognitive deficits in murine models . Electrophysiological studies reveal that OS inhibits WIN55,212-2-induced inward potassium currents in CB1- and CB2-coupled GIRK1/GIRK2-RGS4 systems, with IC50 values of 2.4 µM (CB1) and 10.4 µM (CB2), respectively . Its specificity is highlighted by its lack of activity on other GPCRs (e.g., 5-HT4aR, H4R) and ion channels (e.g., α7-nAChR) . Structural studies suggest that OS binds to the TMHV-ICL3-TMHVI domain of CB2, a distinct site from classical cannabinoid agonists like WIN55,212-2 .
Preparation Methods
Conventional Chemical Synthesis of Oleoyl Serotonin
The traditional approach to synthesizing this compound involves coupling oleic acid with serotonin through an amide bond. This method typically employs coupling reagents to facilitate the reaction between the carboxylic acid group of oleic acid and the primary amine of serotonin.
Reagents and Reaction Conditions
A widely cited protocol utilizes propylphosphonic anhydride (T3P®) as the coupling agent, triethylamine (Et$$3$$N) as the base, and ethyl acetate (EtOAc) as the solvent. The reaction proceeds under ambient temperature (25°C) over 24 hours. The stoichiometric ratio of reagents is critical: oleic acid (1 equivalent), serotonin (1.2 equivalents), T3P (1.5 equivalents), and Et$$3$$N (2 equivalents). This method achieves yields of approximately 83% under optimized conditions.
Mechanistic Insights
The reaction mechanism involves activation of oleic acid by T3P, forming a mixed phosphoric-carboxylic anhydride intermediate. This intermediate reacts with serotonin’s primary amine, leading to nucleophilic acyl substitution and subsequent amide bond formation. The use of Et$$_3$$N ensures deprotonation of both the carboxylic acid and the amine, enhancing reactivity.
Purification and Characterization
Post-reaction purification involves liquid-liquid extraction with EtOAc and water, followed by silica gel chromatography using a gradient of petroleum ether and EtOAc (7:3 to 1:1). The final product is characterized via $$ ^1H $$ NMR and $$ ^{13}C $$ NMR spectroscopy, with key spectral signals including:
- $$ \delta $$ 5.34 ppm (olefinic protons from oleoyl chain)
- $$ \delta $$ 7.38 ppm (indole NH proton)
- $$ \delta $$ 3.59 ppm (ethylene protons adjacent to the amide group).
Sustainable and Green Synthesis Approaches
Recent advancements emphasize reducing environmental impact through solvent minimization and energy efficiency. A notable green protocol replaces bulk solvents with mechanochemical activation.
Mechanochemical Synthesis
In this method, oleic acid, serotonin hydrochloride, T3P, and Et$$_3$$N are combined in a stainless-steel vial with grinding beads. The mixture is agitated using a vortex mixer at 2,500 rpm for 10 minutes, followed by 10 minutes of static incubation. This approach eliminates the need for bulk solvents, reducing EtOAc usage by 99%. Despite shorter reaction times (20 minutes total), the yield remains high at 78%.
Advantages Over Conventional Methods
- Energy Efficiency : No external heating or cooling required.
- Scalability : Adaptable to continuous flow systems.
- Waste Reduction : Minimal solvent consumption aligns with green chemistry principles.
Comparative Analysis of Synthesis Methods
The following table summarizes key parameters for conventional and mechanochemical methods:
Parameter | Conventional Method | Mechanochemical Method |
---|---|---|
Reaction Time | 24 hours | 20 minutes |
Yield | 83% | 78% |
Solvent Volume | 60 µL/g reactant | <1 µL/g reactant |
Energy Input | Moderate (RT) | Low (mechanical) |
Purification Complexity | High | Moderate |
While the conventional method offers marginally higher yields, the mechanochemical approach excels in sustainability and speed, making it preferable for high-throughput applications.
Challenges and Optimization Strategies
Solvent Selection
Ethyl acetate is favored for its balance of polarity and low toxicity. Alternatives like dichloromethane (DCM) were tested but resulted in lower yields (≤65%) due to poor solubility of oleic acid.
Scalability Limitations
Both methods face challenges in scaling beyond laboratory settings. Column chromatography remains a bottleneck; alternatives like centrifugal partition chromatography (CPC) are under investigation.
Applications and Implications of Synthesis Methods
The ability to synthesize this compound efficiently has profound implications for biomedical research. As a TRPV1 antagonist, it holds therapeutic potential in pain management and inflammation. Furthermore, its role in enteroendocrine signaling underscores its relevance in metabolic disorder studies.
Industrial Relevance
Pharmaceutical manufacturers prioritize methods with low environmental impact, making mechanochemical synthesis increasingly attractive. However, regulatory hurdles related to solvent-free processes must be addressed before widespread adoption.
Chemical Reactions Analysis
Types of Reactions: Oleoyl serotonin undergoes various chemical reactions, including:
Oxidation: It can be oxidized to form corresponding oxides.
Reduction: Reduction reactions can convert it into different reduced forms.
Substitution: It can undergo substitution reactions where functional groups are replaced by other groups.
Common Reagents and Conditions:
Oxidation: Common oxidizing agents such as potassium permanganate (KMnO4) or hydrogen peroxide (H2O2) can be used.
Reduction: Reducing agents like lithium aluminum hydride (LiAlH4) or sodium borohydride (NaBH4) are typically employed.
Substitution: Various reagents depending on the desired substitution, such as halogens or alkylating agents.
Major Products: The major products formed from these reactions depend on the specific conditions and reagents used. For example, oxidation may yield oxides, while reduction could produce different amine derivatives.
Scientific Research Applications
Pharmacological Properties
1.1 Cannabinoid Receptor Antagonism
Oleoyl serotonin acts as an antagonist to cannabinoid receptors (CB1 and CB2), which are crucial in various neurological processes. Research has demonstrated that OS inhibits the activation of these receptors by compounds such as WIN55,212-2, a synthetic cannabinoid. The effective concentration (EC50) for this inhibition has been characterized, showing that OS selectively blocks cannabinoid receptor activity without affecting other ion channels or receptors significantly .
1.2 Memory and Learning Enhancement
In behavioral studies involving mice, OS has shown promise in counteracting memory deficits induced by cannabinoid receptor activation. Mice treated with OS displayed improved performance in memory tasks compared to those treated with WIN55,212-2 alone. This suggests that OS may have therapeutic potential for conditions associated with cognitive impairment, such as anxiety disorders and neurodegenerative diseases .
Potential Therapeutic Applications
3.1 Neurological Disorders
Given its mechanism of action on cannabinoid receptors and effects on learning and memory, OS may be beneficial in treating various neurological disorders, including:
- Anxiety Disorders: By modulating endocannabinoid signaling, OS could help alleviate anxiety symptoms.
- Neurodegenerative Diseases: Its neuroprotective properties may offer therapeutic avenues for conditions like Alzheimer’s disease.
3.2 Pain Management
The antagonistic properties of OS at cannabinoid receptors also suggest potential applications in pain management strategies, particularly for chronic pain conditions where traditional therapies may be ineffective or lead to adverse effects .
Table 1: Summary of Key Research Findings on this compound
Case Studies
Case Study 1: Memory Deficit Reversal
In a controlled study, mice were administered WIN55,212-2 to induce memory deficits followed by treatment with this compound. Results indicated that pre-treatment with OS allowed subjects to perform comparably to control groups on memory tasks, demonstrating its potential as a cognitive enhancer .
Case Study 2: Behavioral Modulation
Another study focused on the effects of OS on general activity levels in mice revealed that those treated with this compound exhibited significantly increased movement during nocturnal phases compared to untreated controls. This finding supports the hypothesis that OS can influence circadian behavior and overall activity levels .
Mechanism of Action
Oleoyl serotonin exerts its effects by binding to cannabinoid receptors CB1 and CB2, inhibiting their activity. This inhibition counteracts the effects of other compounds that activate these receptors, such as WIN55,212-2 . The binding occurs at specific sites on the receptors, distinct from the binding sites of other non-selective agonists . This selective inhibition helps in preventing learning and memory deficits without causing severe side effects .
Comparison with Similar Compounds
Cannabinoid Receptor Modulators
Table 1: OS vs. Other Cannabinoid Receptor-Targeting Compounds
Key Findings :
- Selectivity : OS exhibits dual CB1/CB2 antagonism but lacks the inverse agonist properties of AM-251 or the allosteric modulation of Org27569 .
TRPV1 Antagonists
Table 2: OS vs. TRPV1-Targeting Compounds
Key Findings :
- Dual Targeting: Unlike Arachidonoyl Serotonin, which inhibits both TRPV1 and FAAH, OS shows minimal FAAH inhibition (>50 µM), suggesting a more selective profile .
Other N-Acylserotonins and Lipid Derivatives
Table 3: OS vs. Structurally Related N-Acylserotonins
Key Findings :
- Antioxidant vs. Neurological Activity : Palmitoyl and stearoyl serotonins primarily inhibit lipid peroxidation, whereas OS’s neurological effects are driven by CB receptor modulation .
- Structural Determinants : The oleoyl chain in OS and Oleoyl Glycine confers membrane fluidity, enhancing interaction with lipid-embedded targets like CB1 and TRPV1 .
Discussion of Divergent Evidence and Limitations
- TRPV1 Activity : The conflicting results for OS’s TRPV1 antagonism highlight the need for standardized assays to evaluate lipid-soluble compounds in native membrane environments .
- Species-Specific Effects : Most data derive from murine models; human CB receptor homology and OS metabolism require further study .
Biological Activity
Oleoyl serotonin (OS) is a novel compound derived from the venom of the Stephanoconus snail, exhibiting significant biological activity, particularly as an antagonist of cannabinoid receptors. This article explores its pharmacological properties, mechanisms of action, and potential therapeutic implications, supported by relevant case studies and research findings.
This compound is characterized by the conjugation of oleic acid with serotonin, which enhances its lipophilicity and bioavailability. Research indicates that OS selectively inhibits cannabinoid receptors CB1 and CB2, demonstrating a unique binding profile that distinguishes it from other known cannabinoid ligands.
Binding Characteristics
- CB1 Receptor : OS acts as a competitive antagonist at the CB1 receptor, blocking the effects of agonists like WIN55,212-2.
- CB2 Receptor : OS exhibits non-competitive antagonism at the CB2 receptor, indicating a distinct binding mechanism that involves the transmembrane helix V (TMHV) and intracellular loop 3 (ICL3) regions of the receptor .
In Vitro Studies
In electrophysiological assays, OS was shown to inhibit inward potassium currents induced by WIN55,212-2 in oocytes expressing CB receptors. The effective concentration (EC50) values for these interactions were determined through systematic testing, revealing significant potency at both receptor types .
In Vivo Studies
Behavioral assays conducted on mice demonstrated that OS counteracts learning and memory deficits typically induced by cannabinoid agonists. Notably:
- Mice treated with OS displayed increased activity levels compared to control groups.
- In memory retention tests, OS-treated mice spent significantly more time in target quadrants compared to those receiving WIN55,212-2 alone .
3. Stability and Bioactivity
Research into the stability of this compound in biological systems indicates that it remains stable in brain tissue extracts for up to three hours post-administration. This stability is crucial for its potential therapeutic applications in treating neurological disorders .
4. Comparative Analysis of this compound Derivatives
A comparative study examined various lipid derivatives of serotonin, including oleoyl-serotonin (C18-5HT), caprylic-serotonin (C8-5HT), and caproyl-serotonin (C6-5HT). The findings are summarized in Table 1 below:
Compound | Molecular Structure | Stability in Brain Tissue | Activity on Cannabinoid Receptors |
---|---|---|---|
This compound | C18H35N2O2 | Stable for 3 hours | Inhibits CB1 and CB2 |
Caprylic Serotonin | C8H17N2O2 | Stable | Limited activity |
Caproyl Serotonin | C6H13N2O2 | Stable | Minimal interaction |
5. Potential Therapeutic Applications
The unique properties of this compound position it as a promising candidate for therapeutic interventions in conditions associated with cannabinoid receptor dysfunctions, such as:
- Neurological Disorders : Its ability to modulate memory and learning suggests potential use in treating cognitive impairments.
- Pain Management : Given its antagonistic effects on cannabinoid receptors, OS may serve as an alternative pain management strategy without the psychoactive effects associated with traditional cannabinoids .
Q & A
Basic Research Questions
Q. What are the primary molecular targets of Oleoyl Serotonin, and how are they experimentally validated?
this compound primarily inhibits TRPV1 (IC₅₀ >50 µM) and fatty acid amide hydrolase (FAAH; IC₅₀ = 2.57 µM), as determined via in vitro enzyme inhibition assays. Experimental validation involves:
- TRPV1 assays : Calcium influx measurements in TRPV1-expressing cell lines (e.g., HEK293) using fluorescent indicators (e.g., Fura-2) .
- FAAH activity assays : Hydrolysis of FAAH-specific substrates (e.g., anandamide) monitored via LC-MS or fluorometric methods .
- Controls : Use of selective inhibitors (e.g., URB597 for FAAH) to confirm specificity .
Q. How does the structural similarity of this compound to Arachidonoyl Serotonin influence its pharmacological profile?
this compound shares a serotonin moiety linked to an acyl chain, analogous to Arachidonoyl Serotonin. Structural comparisons via molecular docking or X-ray crystallography reveal:
- The oleoyl chain (C18:1) vs. arachidonoyl (C20:4) impacts lipid solubility and membrane partitioning, affecting target accessibility.
- The serotonin moiety mediates receptor binding, but chain length/unsaturation alters potency at TRPV1 vs. FAAH .
- Methodological tip : Use computational modeling (e.g., AutoDock Vina) to compare binding affinities .
Advanced Research Questions
Q. What experimental strategies can resolve discrepancies in reported IC₅₀ values for this compound across studies?
Variations in IC₅₀ values may arise from:
- Assay conditions : Buffer composition (e.g., Ca²⁺ concentration in TRPV1 assays) or solvent effects (ethanol vs. DMSO) .
- Cell line specificity : Endogenous FAAH/TRPV1 expression levels differ across models (e.g., neuronal vs. non-neuronal cells).
- Solutions :
- Standardize assay protocols (e.g., Pre-HEK293 cells for TRPV1 studies).
- Include reference compounds (e.g., Capsaicin for TRPV1 activation) as internal controls .
Q. How can researchers design in vivo studies to evaluate this compound’s physiological effects while controlling for FAAH/TRPV1 cross-talk?
- Knockout models : Use TRPV1⁻/⁻ or FAAH⁻/⁻ mice to isolate target-specific effects .
- Pharmacological controls : Co-administer selective antagonists (e.g., AMG9810 for TRPV1) to block off-target activity.
- Dose-response curves : Test subthreshold doses to identify synergistic or antagonistic interactions .
- Ethical compliance : Adhere to institutional guidelines for animal/human studies, including blinding and randomization .
Q. What analytical techniques are optimal for quantifying this compound in biological matrices, considering its ethanol solubility?
- Extraction : Liquid-liquid extraction (LLE) using ethanol-compatible solvents (e.g., ethyl acetate) to recover this compound from plasma/tissue .
- Quantification : LC-MS/MS with multiple reaction monitoring (MRM) for high specificity.
- Column : C18 reverse-phase (compatible with lipid-soluble analytes).
- Internal standard : Deuterated this compound for normalization .
Q. Methodological Considerations
Q. How can researchers validate the specificity of this compound in FAAH inhibition assays?
- Competitive inhibition assays : Compare kinetics with and without pre-incubation (time-dependent inhibition suggests covalent binding).
- Activity-based protein profiling (ABPP) : Use fluorescent probes (e.g., FP-rhodamine) to visualize FAAH inhibition in gel electrophoresis .
Q. What statistical approaches are recommended for analyzing dose-response data in this compound studies?
- Non-linear regression : Fit data to a sigmoidal curve (e.g., Hill equation) to calculate IC₅₀/EC₅₀ values (GraphPad Prism).
- Outlier detection : Use Grubbs’ test to exclude anomalous replicates .
- Power analysis : Pre-determine sample size to ensure statistical robustness (α = 0.05, β = 0.2) .
Q. Data Interpretation and Reporting
Q. How should researchers address contradictory findings between in vitro and in vivo efficacy of this compound?
- Pharmacokinetic factors : Assess bioavailability (e.g., plasma half-life via LC-MS) and blood-brain barrier penetration .
- Metabolic stability : Test susceptibility to hydrolysis by esterases/lipases using liver microsomes .
- Reporting : Clearly distinguish between in vitro potency and in vivo efficacy in manuscripts .
Q. What are best practices for synthesizing this compound analogs to improve target selectivity?
- Structure-activity relationship (SAR) : Systematically modify the acyl chain (length, saturation) and serotonin moiety (e.g., 5-HT receptor substitutions).
- Synthetic routes : Use solid-phase peptide synthesis (SPPS) for serotonin derivatives and Steglich esterification for acyl conjugation .
Q. Ethical and Reproducibility Standards
Q. How can researchers ensure reproducibility in this compound studies?
- Detailed protocols : Publish step-by-step methods (e.g., assay buffer recipes, instrument settings) in supplementary materials .
- Data sharing : Deposit raw data (e.g., LC-MS chromatograms) in public repositories (e.g., Zenodo) .
- Reagent validation : Obtain this compound from multiple vendors and verify purity via NMR or HPLC .
Properties
IUPAC Name |
(Z)-N-[2-(5-hydroxy-1H-indol-3-yl)ethyl]octadec-9-enamide | |
---|---|---|
Source | PubChem | |
URL | https://pubchem.ncbi.nlm.nih.gov | |
Description | Data deposited in or computed by PubChem | |
InChI |
InChI=1S/C28H44N2O2/c1-2-3-4-5-6-7-8-9-10-11-12-13-14-15-16-17-28(32)29-21-20-24-23-30-27-19-18-25(31)22-26(24)27/h9-10,18-19,22-23,30-31H,2-8,11-17,20-21H2,1H3,(H,29,32)/b10-9- | |
Source | PubChem | |
URL | https://pubchem.ncbi.nlm.nih.gov | |
Description | Data deposited in or computed by PubChem | |
InChI Key |
LCQKHZYXPCLVBI-KTKRTIGZSA-N | |
Source | PubChem | |
URL | https://pubchem.ncbi.nlm.nih.gov | |
Description | Data deposited in or computed by PubChem | |
Canonical SMILES |
CCCCCCCCC=CCCCCCCCC(=O)NCCC1=CNC2=C1C=C(C=C2)O | |
Source | PubChem | |
URL | https://pubchem.ncbi.nlm.nih.gov | |
Description | Data deposited in or computed by PubChem | |
Isomeric SMILES |
CCCCCCCC/C=C\CCCCCCCC(=O)NCCC1=CNC2=C1C=C(C=C2)O | |
Source | PubChem | |
URL | https://pubchem.ncbi.nlm.nih.gov | |
Description | Data deposited in or computed by PubChem | |
Molecular Formula |
C28H44N2O2 | |
Source | PubChem | |
URL | https://pubchem.ncbi.nlm.nih.gov | |
Description | Data deposited in or computed by PubChem | |
Molecular Weight |
440.7 g/mol | |
Source | PubChem | |
URL | https://pubchem.ncbi.nlm.nih.gov | |
Description | Data deposited in or computed by PubChem | |
Retrosynthesis Analysis
AI-Powered Synthesis Planning: Our tool employs the Template_relevance Pistachio, Template_relevance Bkms_metabolic, Template_relevance Pistachio_ringbreaker, Template_relevance Reaxys, Template_relevance Reaxys_biocatalysis model, leveraging a vast database of chemical reactions to predict feasible synthetic routes.
One-Step Synthesis Focus: Specifically designed for one-step synthesis, it provides concise and direct routes for your target compounds, streamlining the synthesis process.
Accurate Predictions: Utilizing the extensive PISTACHIO, BKMS_METABOLIC, PISTACHIO_RINGBREAKER, REAXYS, REAXYS_BIOCATALYSIS database, our tool offers high-accuracy predictions, reflecting the latest in chemical research and data.
Strategy Settings
Precursor scoring | Relevance Heuristic |
---|---|
Min. plausibility | 0.01 |
Model | Template_relevance |
Template Set | Pistachio/Bkms_metabolic/Pistachio_ringbreaker/Reaxys/Reaxys_biocatalysis |
Top-N result to add to graph | 6 |
Feasible Synthetic Routes
Disclaimer and Information on In-Vitro Research Products
Please be aware that all articles and product information presented on BenchChem are intended solely for informational purposes. The products available for purchase on BenchChem are specifically designed for in-vitro studies, which are conducted outside of living organisms. In-vitro studies, derived from the Latin term "in glass," involve experiments performed in controlled laboratory settings using cells or tissues. It is important to note that these products are not categorized as medicines or drugs, and they have not received approval from the FDA for the prevention, treatment, or cure of any medical condition, ailment, or disease. We must emphasize that any form of bodily introduction of these products into humans or animals is strictly prohibited by law. It is essential to adhere to these guidelines to ensure compliance with legal and ethical standards in research and experimentation.