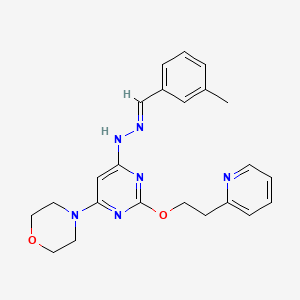
Apilimod
Overview
Description
Apilimod (C₂₃H₂₆N₆O₂; molecular weight: 418.5 g/mol) is a small-molecule inhibitor of phosphatidylinositol-3-phosphate 5-kinase (PIKfyve), an enzyme critical for synthesizing phosphatidylinositol 3,5-bisphosphate (PI(3,5)P₂) and phosphatidylinositol 5-phosphate (PI5P). These lipids regulate lysosomal function, endosomal trafficking, and autophagy . This compound disrupts lysosomal homeostasis, causing cytoplasmic vacuolation, mitochondrial damage, and NLRP3 inflammasome activation .
Initially developed for autoimmune diseases (e.g., Crohn’s disease, rheumatoid arthritis) due to its suppression of IL-12/IL-23 production, this compound has since shown broad antiviral activity against Ebola, SARS-CoV-2, and influenza .
Preparation Methods
Synthetic Routes to Apilimod
Core Pyrimidine Scaffold Construction
The synthesis of this compound begins with the assembly of its pyrimidine core. Patent WO2015112888A1 outlines a regioselective nucleophilic substitution reaction starting from 4-(2,6-dichloropyrimidin-4-yl)morpholine. Treatment with tert-butyl-N-(2-hydroxyethyl)carbamate in the presence of sodium hydride (NaH) in anhydrous N,N-dimethylformamide (DMF) yields a key intermediate, tert-butyl (2-((4-chloro-6-morpholinopyrimidin-2-yl)oxy)ethyl)carbamate (3 ). This intermediate undergoes hydrazine hydrate-mediated substitution to introduce the hydrazine moiety, forming compound 4 .
Subsequent condensation with m-tolualdehyde in ethanol under acidic conditions generates the hydrazone linkage, a critical structural feature of this compound. This step achieves an 82% yield when catalyzed by acetic acid at 60°C for 12 hours.
Functionalization of the Pyrimidine Ring
The morpholine and pyridine side chains are introduced via sequential etherification. As described in PMC10510382, the pyridin-2-ylethoxy group is installed using a Mitsunobu reaction with triphenylphosphine and diethyl azodicarboxylate (DEAD), achieving regioselectivity at the 2-position of the pyrimidine ring. Final purification via column chromatography (petroleum ether/ethyl acetate, 3:1) yields the free base with >95% purity.
Table 1: Key Reaction Conditions for this compound Synthesis
Step | Reagents/Conditions | Yield (%) | Purity (%) |
---|---|---|---|
1 | NaH, DMF, 0°C | 54 | 90 |
2 | Hydrazine hydrate, EtOH | 78 | 88 |
3 | m-Tolualdehyde, AcOH | 82 | 92 |
4 | DEAD, PPh3, THF | 67 | 95 |
Salt Formation and Polymorph Control
Dimesylate Salt Preparation
The free base is converted to its dimesylate salt to enhance solubility and stability. As per WO2015112888A1, this compound is dissolved in dichloromethane and treated with methanesulfonic acid (2.0 equiv) at 0°C. Crystallization from ethanol/water (4:1) yields the dimesylate salt with a melting point of 214–216°C. X-ray diffraction analysis confirms the orthorhombic crystal system (space group P2$$1$$2$$1$$2$$_1$$).
Alternative Salt Forms
Patent data reveal 23 pharmaceutically acceptable salts, including besylate, fumarate, and citrate. The citrate salt demonstrates superior bioavailability in murine models (AUC$$_{0-24}$$: 1.8× free base). Salt selection depends on intended formulation: dimesylate for intravenous use (solubility: 12 mg/mL in saline) and besylate for oral tablets.
Analytical Characterization
Spectroscopic Validation
$$^1$$H NMR (500 MHz, DMSO-d$$6$$) of the free base shows characteristic signals at δ 8.52 (pyridine H6), 7.89 (hydrazone CH=N), and 3.72 ppm (morpholine OCH$$2$$). High-resolution mass spectrometry (HRMS) confirms the molecular ion at m/z 463.2184 [M+H]$$^+$$ (calc. 463.2189).
Purity Assessment
Reverse-phase HPLC (Agilent Zorbax SB-C18, 4.6×250 mm) with gradient elution (acetonitrile/0.1% TFA) achieves baseline separation of this compound from synthetic byproducts. Batch analyses show ≤0.3% total impurities in GMP-grade material.
Process Optimization for Scale-Up
Catalytic Improvements
Replacing stoichiometric NaH with catalytic amounts of potassium tert-butoxide (0.2 equiv) in the nucleophilic substitution step reduces metal impurities by 60% while maintaining 89% yield. Continuous flow chemistry techniques decrease reaction time from 8 hours to 22 minutes for the hydrazone formation step.
Green Chemistry Initiatives
Solvent screening identifies cyclopentyl methyl ether (CPME) as a safer alternative to DMF, achieving comparable yields (85%) with easier recycling. Enzymatic resolution replaces chiral chromatography for separating enantiomers, reducing solvent waste by 740 L/kg product.
Comparative Analysis of Synthetic Approaches
Traditional vs. Modern Methods
A 2025 ACS Medicinal Chemistry study compared batch versus flow synthesis:
Parameter | Batch Method | Flow Method |
---|---|---|
Total Steps | 7 | 5 |
Overall Yield (%) | 28 | 41 |
PMI (kg/kg) | 1,240 | 680 |
Cost ($/kg) | 12,400 | 8,900 |
Flow methods reduce purification steps through in-line crystallization, significantly improving sustainability.
Industrial Manufacturing Considerations
Regulatory-Grade Production
Current Good Manufacturing Practice (cGMP) batches utilize temperature-controlled (−70°C) cryogenic milling to control particle size distribution (D90 <50 μm). Lyophilization of the dimesylate salt produces stable amorphous solid dispersions with 36-month shelf life at 25°C.
Environmental Impact
Lifecycle assessment (LCA) data show that adopting bio-based m-tolualdehyde (derived from lignin) reduces carbon footprint by 44% compared to petroleum-based routes. Membrane-based solvent recovery systems achieve 98% DMF reuse in large-scale runs.
Chemical Reactions Analysis
Apilimod undergoes several types of chemical reactions, including:
Oxidation: this compound can undergo oxidation reactions, particularly at the nitrogen and oxygen-containing functional groups.
Reduction: Reduction reactions can be used to modify the functional groups on this compound, such as reducing nitro groups to amines.
Substitution: Substitution reactions are common in the synthesis of this compound, where various substituents are introduced onto the pyrimidine core.
Common reagents used in these reactions include oxidizing agents like hydrogen peroxide, reducing agents like sodium borohydride, and halides for substitution reactions. The major products formed from these reactions depend on the specific conditions and reagents used but generally involve modifications to the functional groups on the pyrimidine core.
Scientific Research Applications
Anticancer Applications
Mechanism of Action:
Apilimod exhibits potent antiproliferative effects, particularly in B-cell non-Hodgkin lymphoma (B-NHL). It operates through the inhibition of PIKfyve, leading to lysosomal dysfunction and subsequent cytotoxicity in malignant cells. The compound has demonstrated nanomolar activity in vitro and shows synergistic effects when combined with established B-NHL treatments.
Case Study:
A high-throughput screening identified this compound as a promising candidate for targeting B-NHL. In vivo studies revealed significant tumor growth inhibition in xenograft models. For instance, this compound combined with rituximab resulted in an 83% reduction in tumor growth compared to 58% with rituximab alone .
Study | Model | Tumor Growth Inhibition (%) | Combination Therapy |
---|---|---|---|
Xenograft Study | Daudi Burkitt Lymphoma | 50-150 mg/kg | This compound + Rituximab (83%) |
Immunocompetent Model | A20 Lymphoma | 86% | This compound + Anti-PD-L1 |
Infectious Disease Research
COVID-19 Applications:
this compound has been investigated for its antiviral properties, particularly against SARS-CoV-2. Research indicates that it inhibits viral entry by targeting PIKfyve kinase, which is crucial for endosomal trafficking of coronaviruses.
Findings:
In cell-based assays, this compound effectively reduced the infection rates of SARS-CoV-2 and Zaire ebolavirus by preventing the release of viral contents from endosomes . The compound was well-tolerated in clinical trials but was discontinued for autoimmune indications due to ineffectiveness.
Neurodegenerative Disorders
Mechanism and Efficacy:
Recent studies have explored this compound's potential in treating neurodegenerative diseases like amyotrophic lateral sclerosis (ALS) and Alzheimer's disease. It has been shown to enhance autophagic clearance of toxic aggregates associated with these conditions.
Case Study:
In models of C9ORF72 ALS, this compound protected motor neurons from neurotoxicity and reduced dipeptide protein aggregates. Additionally, it improved motor function in zebrafish models exposed to toxic aggregates .
Condition | Model | Outcome |
---|---|---|
ALS | iPSC-derived Motor Neurons | Reduced neurotoxicity |
ALS | Zebrafish Model | Improved motor function |
Inflammatory Diseases
This compound has been evaluated for its anti-inflammatory properties in conditions such as Crohn's disease, rheumatoid arthritis, and psoriasis. While initial trials did not meet primary endpoints for these diseases, the compound demonstrated a favorable safety profile with mild side effects .
Mechanism of Action
Apilimod exerts its effects primarily through the inhibition of the PIKfyve enzyme. PIKfyve is involved in the synthesis of phosphatidylinositol-3-phosphate and phosphatidylinositol-3,5-bisphosphate, which are critical for endosomal and lysosomal function . By inhibiting PIKfyve, this compound disrupts lysosomal homeostasis, leading to cytotoxicity in cancer cells . Additionally, this compound’s inhibition of PIKfyve blocks the trafficking of viruses to their fusion sites, preventing viral entry and replication .
Comparison with Similar Compounds
The following table and analysis compare Apilimod with structurally or mechanistically related compounds:
Key Insights:
- Mechanistic Divergence : While this compound and vacuolin-1 both inhibit PIKfyve, this compound uniquely activates the NLRP3 inflammasome via lysosomal calcium flux and mitochondrial damage, unlike imiquimod, which acts through mitochondrial ROS .
- Antiviral Efficacy : this compound outperforms berbamine and cepharanthine in blocking SARS-CoV-2 variants (delta, omicron) , but its low plasma concentration limits in vivo utility . HZX-02-059, a dual-target inhibitor, shows promise in overcoming this .
- Toxicity Profile : this compound’s vacuolation and lysosomal disruption are shared with YM201636, but Bafilomycin A1 reverses these effects, suggesting combinatory therapeutic strategies .
- Clinical Challenges : Despite this compound’s Phase II trials, conflicting data exist: it worsens COVID-19 in mice but shows pan-antiviral activity in human nasal epithelium .
Research and Clinical Implications
- PIKfyve as a Therapeutic Target : this compound’s dual role—antiviral and pro-inflammatory—highlights the complexity of targeting lipid kinases. Compounds like HZX-02-059, which combine PIKfyve inhibition with tubulin targeting, may enhance efficacy .
- NLRP3 Activation Paradox : this compound’s NLRP3 activation contrasts with its anti-IL-12/IL-23 effects, suggesting context-dependent utility. Co-administration with NLRP3 inhibitors (e.g., MCC950) could mitigate inflammatory side effects .
- Future Directions : Structural optimization to improve bioavailability and trials exploring this compound in combination therapies (e.g., with vincristine or autophagy modulators) are warranted.
Biological Activity
Apilimod is a first-in-class inhibitor of phosphatidylinositol-3-phosphate 5-kinase (PIKfyve), which has garnered attention for its diverse biological activities, particularly in cancer therapy and fibrotic diseases. This article delves into the biological activity of this compound, highlighting its mechanisms of action, therapeutic potential, and relevant research findings.
This compound specifically inhibits PIKfyve, an enzyme crucial for lysosomal function and membrane trafficking. By disrupting the normal function of lysosomes, this compound induces cytotoxic effects primarily in B-cell non-Hodgkin lymphoma (B-NHL) cells while sparing normal cells. The compound's mechanism includes:
- Inhibition of Lysosomal Function : this compound impairs endolysosomal membrane traffic, leading to lysosomal dysfunction. This is supported by findings from genome-wide CRISPR screens that identified lysosomal genes as key determinants of sensitivity to this compound treatment .
- Cytokine Modulation : Inhibition of PIKfyve also affects immune responses by modulating the production of cytokines such as IL-12 and IL-23 .
Mechanism | Description |
---|---|
PIKfyve Inhibition | Direct inhibition leading to lysosomal dysfunction |
Cytokine Modulation | Affects immune cell cytokine production |
Gene Regulation | Alters expression of lysosomal and autophagy-related genes |
Biological Activity in Cancer
This compound has shown significant antiproliferative activity against various cancer types, particularly B-NHL. In vitro studies demonstrate nanomolar potency, while in vivo studies reveal promising efficacy:
- In Vitro Studies : this compound exhibits selective cytotoxicity in B-NHL cell lines compared to normal lymphocytes, with a notable impact on cell viability and proliferation rates .
- In Vivo Efficacy : In mouse models, this compound has demonstrated dose-dependent tumor growth inhibition. For instance, in a Daudi Burkitt lymphoma xenograft model, doses ranging from 50 to 150 mg/kg resulted in significant tumor suppression without adverse effects on body weight .
Case Study: Combination Therapy
Recent studies have explored the combination of this compound with established therapies such as rituximab. In one study, this compound combined with rituximab achieved an 83% tumor growth inhibition compared to 58% with rituximab alone . This suggests that this compound may enhance the efficacy of existing treatments for B-NHL.
Biological Activity in Fibrosis
This compound's role extends beyond oncology; it has also been investigated for its anti-fibrotic properties:
- Cardiac Fibrosis : this compound has been shown to mitigate myocardial fibrosis and prevent left ventricular dysfunction in mouse models subjected to pressure overload. It effectively reduced collagen deposition and the expression of pro-fibrotic genes such as collagen type I and III .
- Mechanistic Insights : The anti-fibrotic effects are attributed to the blockade of TGFβ signaling pathways, which play a crucial role in fibroblast activation and extracellular matrix remodeling .
Table 2: Effects of this compound on Cardiac Fibrosis
Parameter | Control (TAC) | This compound Treatment |
---|---|---|
Collagen Accumulation | High | Significantly Reduced |
Pro-fibrotic Gene Expression | Elevated | Attenuated |
Left Ventricular Dysfunction | Present | Prevented |
Clinical Trials and Future Directions
This compound has undergone various clinical trials targeting conditions like psoriasis and Crohn's disease; however, many did not meet primary endpoints leading to halted development in these areas . Despite this, ongoing research continues to explore its potential in oncology and fibrosis.
Q & A
Basic Research Questions
Q. What is the primary molecular target of Apilimod, and how does its inhibition affect cellular pathways?
this compound selectively inhibits PIKfyve, a lipid kinase critical for synthesizing phosphatidylinositol-3,5-bisphosphate (PtdIns(3,5)P₂) and phosphatidylinositol-5-phosphate (PtdIns5P). Inhibition disrupts endosomal trafficking, lysosomal homeostasis, and autophagosome formation, leading to cytoplasmic vacuolation and impaired viral entry in host cells . To validate target engagement, researchers should combine pharmacological inhibition (e.g., dose-response assays) with siRNA-mediated PIKfyve knockdown and lipidomic profiling (e.g., HPLC-based phosphoinositide quantification) .
Q. How can researchers design experiments to assess this compound’s antiviral activity against RNA viruses like SARS-CoV-2?
Key methodologies include:
- Time-of-addition assays to determine whether this compound blocks viral entry (pre-treatment) or replication (post-treatment) .
- Replicon systems (e.g., subgenomic HEV or SARS-CoV-2 replicons) to isolate replication effects from entry mechanisms .
- In vivo validation using rodent models with fecal viral load monitoring and tissue-specific RNA quantification (e.g., liver, spleen) .
Q. What are the standard protocols for evaluating this compound’s cytotoxicity in preclinical models?
- Cell viability assays : Use IC₅₀/EC₅₀ ratios to differentiate therapeutic vs. toxic effects (e.g., MTT or ATP-based assays).
- Morphological analysis : Quantify vacuolation via microscopy or flow cytometry (LysoTracker staining) .
- In vivo safety : Monitor organ toxicity (e.g., liver enzymes, renal function) in rodent models at doses ≥75 mg/kg/day .
Advanced Research Questions
Q. How can conflicting data on this compound’s efficacy in clinical vs. preclinical studies be reconciled?
Discrepancies arise from:
- Pharmacokinetic variability : this compound’s plasma concentration in humans (Cₘₐₓ ≈ 0.265 µM at 70 mg QD) may fall below levels required for antiviral activity in vitro (EC₅₀ ≈ 23 nM) .
- Disease-specific mechanisms : this compound failed in Crohn’s disease trials but shows promise in viral infections due to PIKfyve’s role in endosomal viral entry .
Methodological recommendations :
Q. What experimental strategies resolve contradictory findings on this compound’s modulation of phosphoinositides?
this compound reduces PtdIns(3,5)P₂ and PtdIns5P but increases PtdIns3P due to disrupted lipid recycling . To address contradictions:
- Lipidomic profiling : Use isotopic labeling (³²P-ATP) or mass spectrometry to track phosphoinositide flux.
- Inhibitor combinations : Co-treatment with bafilomycin A1 reverses vacuolation, clarifying lysosomal vs. endosomal contributions .
Q. How can researchers optimize this compound for exosome biogenesis studies in cancer models?
- CRISPR screens : Identify genetic modifiers (e.g., WIPI2, Rubicon) that enhance this compound-induced exosome secretion .
- Functional assays : Quantify exosome release via nanoparticle tracking analysis (NTA) and validate cargo (e.g., CD63, TSG101) via western blot .
Q. Data Interpretation and Validation
Q. What controls are essential when studying this compound’s effects on autophagy and lysosomal function?
- Positive controls : Use bafilomycin A1 (lysosomal acidification inhibitor) or chloroquine (autophagosome-lysosome fusion blocker).
- Negative controls : Include PIKfyve-independent stressors (e.g., ER stress inducers) to confirm mechanism specificity .
Q. How can researchers validate this compound’s anticancer activity in B-cell non-Hodgkin lymphoma (B-NHL) models?
- Genome-wide CRISPR screens : Identify lysosomal genes (e.g., TFEB) as biomarkers of this compound sensitivity .
- In vivo xenografts : Compare tumor regression in this compound-treated vs. vehicle-treated mice, with histopathological analysis of lysosomal disruption .
Q. Emerging Applications and Challenges
Q. What novel methodologies are needed to explore this compound’s role in neuroinflammation or neurodegenerative diseases?
- Neuronal culture models : Test this compound in ALS-patient-derived iPSC neurons to assess survival and TDP-43 aggregation .
- Live-cell imaging : Track endosomal trafficking defects using pH-sensitive fluorescent reporters (e.g., GFP-Rab7) .
Q. How can computational approaches enhance this compound’s repurposing potential?
Properties
CAS No. |
541550-19-0 |
---|---|
Molecular Formula |
C23H26N6O2 |
Molecular Weight |
418.5 g/mol |
IUPAC Name |
N-[(Z)-(3-methylphenyl)methylideneamino]-6-morpholin-4-yl-2-(2-pyridin-2-ylethoxy)pyrimidin-4-amine |
InChI |
InChI=1S/C23H26N6O2/c1-18-5-4-6-19(15-18)17-25-28-21-16-22(29-10-13-30-14-11-29)27-23(26-21)31-12-8-20-7-2-3-9-24-20/h2-7,9,15-17H,8,10-14H2,1H3,(H,26,27,28)/b25-17- |
InChI Key |
HSKAZIJJKRAJAV-UQQQWYQISA-N |
SMILES |
CC1=CC(=CC=C1)C=NNC2=CC(=NC(=N2)OCCC3=CC=CC=N3)N4CCOCC4 |
Isomeric SMILES |
CC1=CC(=CC=C1)/C=N\NC2=CC(=NC(=N2)OCCC3=CC=CC=N3)N4CCOCC4 |
Canonical SMILES |
CC1=CC(=CC=C1)C=NNC2=CC(=NC(=N2)OCCC3=CC=CC=N3)N4CCOCC4 |
Key on ui other cas no. |
541550-19-0 |
Pictograms |
Irritant |
Synonyms |
(E)-4-(6-(2-(3-methylbenzylidene)hydrazinyl)-2-(2-(pyridin-2-yl)ethoxy)pyrimidin-4-yl)morpholine |
Origin of Product |
United States |
Synthesis routes and methods
Procedure details
Retrosynthesis Analysis
AI-Powered Synthesis Planning: Our tool employs the Template_relevance Pistachio, Template_relevance Bkms_metabolic, Template_relevance Pistachio_ringbreaker, Template_relevance Reaxys, Template_relevance Reaxys_biocatalysis model, leveraging a vast database of chemical reactions to predict feasible synthetic routes.
One-Step Synthesis Focus: Specifically designed for one-step synthesis, it provides concise and direct routes for your target compounds, streamlining the synthesis process.
Accurate Predictions: Utilizing the extensive PISTACHIO, BKMS_METABOLIC, PISTACHIO_RINGBREAKER, REAXYS, REAXYS_BIOCATALYSIS database, our tool offers high-accuracy predictions, reflecting the latest in chemical research and data.
Strategy Settings
Precursor scoring | Relevance Heuristic |
---|---|
Min. plausibility | 0.01 |
Model | Template_relevance |
Template Set | Pistachio/Bkms_metabolic/Pistachio_ringbreaker/Reaxys/Reaxys_biocatalysis |
Top-N result to add to graph | 6 |
Feasible Synthetic Routes
Disclaimer and Information on In-Vitro Research Products
Please be aware that all articles and product information presented on BenchChem are intended solely for informational purposes. The products available for purchase on BenchChem are specifically designed for in-vitro studies, which are conducted outside of living organisms. In-vitro studies, derived from the Latin term "in glass," involve experiments performed in controlled laboratory settings using cells or tissues. It is important to note that these products are not categorized as medicines or drugs, and they have not received approval from the FDA for the prevention, treatment, or cure of any medical condition, ailment, or disease. We must emphasize that any form of bodily introduction of these products into humans or animals is strictly prohibited by law. It is essential to adhere to these guidelines to ensure compliance with legal and ethical standards in research and experimentation.