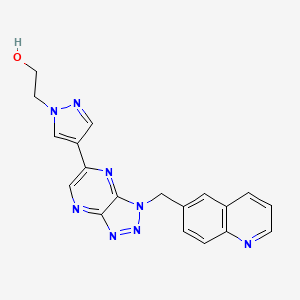
PF-04217903
Overview
Description
PF-04217903 is a novel ATP-competitive small-molecule inhibitor of the c-Met kinase. The c-Met pathway has been implicated in various human cancers due to its critical role in tumor growth, invasion, and metastasis. This compound has shown more than 1,000-fold selectivity for c-Met compared with over 150 kinases, making it one of the most selective c-Met inhibitors described to date .
Preparation Methods
Synthetic Routes and Reaction Conditions: PF-04217903 is synthesized through a series of chemical reactions involving the formation of a quinoline moiety, which consists of a benzene ring fused to a pyrimidine ring to form benzo[b]azabenzene . The synthesis involves multiple steps, including the formation of intermediates and their subsequent reactions under controlled conditions.
Industrial Production Methods: The industrial production of this compound involves large-scale synthesis using optimized reaction conditions to ensure high yield and purity. The process includes the use of specific reagents and catalysts to facilitate the reactions and achieve the desired product.
Chemical Reactions Analysis
Types of Reactions: PF-04217903 undergoes various chemical reactions, including:
Oxidation: The compound can be oxidized under specific conditions to form oxidized derivatives.
Reduction: Reduction reactions can be performed to modify the functional groups present in the compound.
Substitution: Substitution reactions can be carried out to replace specific atoms or groups within the molecule.
Common Reagents and Conditions:
Oxidation: Common oxidizing agents such as hydrogen peroxide or potassium permanganate can be used.
Reduction: Reducing agents like sodium borohydride or lithium aluminum hydride are commonly employed.
Substitution: Substitution reactions often involve the use of halogenating agents or nucleophiles under controlled conditions.
Major Products Formed: The major products formed from these reactions depend on the specific conditions and reagents used. For example, oxidation may yield oxidized derivatives, while reduction can produce reduced forms of the compound.
Scientific Research Applications
PF-04217903 has a wide range of scientific research applications, including:
Chemistry: Used as a tool compound to study the c-Met pathway and its role in various chemical reactions.
Biology: Employed in biological studies to investigate the effects of c-Met inhibition on cellular processes such as proliferation, migration, and invasion
Medicine: Explored for its potential therapeutic applications in treating cancers that exhibit c-Met dysregulation. .
Industry: Utilized in the development of targeted therapies and as a reference compound in drug discovery and development.
Mechanism of Action
PF-04217903 exerts its effects by selectively inhibiting the c-Met kinase. The c-Met receptor tyrosine kinase is frequently mutated or abnormally activated in late-stage human cancers and plays a critical role in tumor progression, invasive growth, and angiogenesis . This compound inhibits c-Met phosphorylation, leading to the suppression of downstream signaling pathways involved in tumor cell proliferation, survival, migration, and invasion . Additionally, it exhibits antiangiogenic properties by reducing tumor microvessel density and plasma levels of vascular endothelial growth factor A and interleukin-8 .
Comparison with Similar Compounds
PF-04217903 is unique due to its high selectivity for c-Met compared with other kinases. Similar compounds include:
Cabozantinib: A multi-kinase inhibitor that targets c-Met and other kinases but lacks the high selectivity of this compound.
Crizotinib: Another c-Met inhibitor with broader kinase inhibition profile compared to this compound.
These comparisons highlight the uniqueness of this compound in terms of its selectivity and potency as a c-Met inhibitor.
Biological Activity
PF-04217903 is a selective, ATP-competitive small-molecule inhibitor of the c-Met receptor tyrosine kinase (RTK), which plays a crucial role in various cancers by regulating processes such as cell proliferation, survival, migration, invasion, and angiogenesis. Its biological activity has been extensively studied, revealing significant potential for therapeutic applications in oncology.
This compound selectively inhibits c-Met with over 1,000-fold selectivity compared to more than 150 other kinases, making it one of the most selective c-Met inhibitors available. The compound demonstrates high affinity with an IC50 value ranging from 3.1 nM to 142 nM against different targets . Upon binding to c-Met, this compound prevents its phosphorylation and subsequent activation of downstream signaling pathways, including MAPK and PI3K/AKT pathways, which are critical for tumor growth and metastasis .
Key Findings from Research Studies
- In Vitro Efficacy : this compound has shown potent inhibition of tumor cell proliferation and survival in MET-amplified cell lines. In studies involving human tumor models, the compound effectively reduced cell migration and invasion .
- In Vivo Efficacy : In xenograft models expressing high levels of c-Met, this compound exhibited dose-dependent antitumor activity. However, complete inhibition of c-Met activity resulted in only partial tumor growth inhibition (38%-46%), suggesting that additional mechanisms may contribute to tumor resistance .
- Combination Therapies : The combination of this compound with other agents has been explored to enhance antitumor efficacy. For instance, combining it with RON shRNA knockdown led to a significant increase in apoptosis and enhanced tumor inhibition compared to either treatment alone .
- Resistance Mechanisms : Prolonged exposure to this compound in certain gastric cancer cell lines resulted in resistance due to the emergence of a novel SND1-BRAF fusion protein that activates the MAPK pathway. This underscores the importance of understanding resistance mechanisms when developing treatment strategies involving c-Met inhibitors .
Biological Activity Summary Table
Parameter | Value |
---|---|
Selectivity | >1000-fold for c-Met over 150 kinases |
IC50 (wild-type c-Met) | 6-7 nM |
IC50 (various mutants) | Varies (specific values not provided) |
Tumor Growth Inhibition | 38%-46% in high c-Met models |
Resistance Mechanism | SND1-BRAF fusion protein |
Case Study 1: Sensitivity in Tumor Models
A study assessed the sensitivity of various human tumor models to this compound. Results indicated that while the compound effectively inhibited tumor growth in MET-amplified models, its efficacy was limited by the presence of alternative oncogenic pathways .
Case Study 2: Resistance Development
In another investigation involving gastric cancer cells (GTL16), prolonged exposure to this compound led to resistance through a chromosomal rearrangement producing a constitutively active SND1-BRAF fusion protein. This case highlights the need for combination therapies to overcome resistance mechanisms .
Properties
IUPAC Name |
2-[4-[3-(quinolin-6-ylmethyl)triazolo[4,5-b]pyrazin-5-yl]pyrazol-1-yl]ethanol | |
---|---|---|
Source | PubChem | |
URL | https://pubchem.ncbi.nlm.nih.gov | |
Description | Data deposited in or computed by PubChem | |
InChI |
InChI=1S/C19H16N8O/c28-7-6-26-12-15(9-22-26)17-10-21-18-19(23-17)27(25-24-18)11-13-3-4-16-14(8-13)2-1-5-20-16/h1-5,8-10,12,28H,6-7,11H2 | |
Source | PubChem | |
URL | https://pubchem.ncbi.nlm.nih.gov | |
Description | Data deposited in or computed by PubChem | |
InChI Key |
PDMUGYOXRHVNMO-UHFFFAOYSA-N | |
Source | PubChem | |
URL | https://pubchem.ncbi.nlm.nih.gov | |
Description | Data deposited in or computed by PubChem | |
Canonical SMILES |
C1=CC2=C(C=CC(=C2)CN3C4=NC(=CN=C4N=N3)C5=CN(N=C5)CCO)N=C1 | |
Source | PubChem | |
URL | https://pubchem.ncbi.nlm.nih.gov | |
Description | Data deposited in or computed by PubChem | |
Molecular Formula |
C19H16N8O | |
Source | PubChem | |
URL | https://pubchem.ncbi.nlm.nih.gov | |
Description | Data deposited in or computed by PubChem | |
DSSTOX Substance ID |
DTXSID401026097 | |
Record name | 2-(4-(1-(Quinolin-6-ylmethyl)-1H-(1,2,3)triazolo(4,5-b)pyrazin-6-yl)-1H-pyrazol-1-yl)ethanol | |
Source | EPA DSSTox | |
URL | https://comptox.epa.gov/dashboard/DTXSID401026097 | |
Description | DSSTox provides a high quality public chemistry resource for supporting improved predictive toxicology. | |
Molecular Weight |
372.4 g/mol | |
Source | PubChem | |
URL | https://pubchem.ncbi.nlm.nih.gov | |
Description | Data deposited in or computed by PubChem | |
CAS No. |
956905-27-4 | |
Record name | PF-04217903 | |
Source | ChemIDplus | |
URL | https://pubchem.ncbi.nlm.nih.gov/substance/?source=chemidplus&sourceid=0956905274 | |
Description | ChemIDplus is a free, web search system that provides access to the structure and nomenclature authority files used for the identification of chemical substances cited in National Library of Medicine (NLM) databases, including the TOXNET system. | |
Record name | PF-04217903 | |
Source | DrugBank | |
URL | https://www.drugbank.ca/drugs/DB12848 | |
Description | The DrugBank database is a unique bioinformatics and cheminformatics resource that combines detailed drug (i.e. chemical, pharmacological and pharmaceutical) data with comprehensive drug target (i.e. sequence, structure, and pathway) information. | |
Explanation | Creative Common's Attribution-NonCommercial 4.0 International License (http://creativecommons.org/licenses/by-nc/4.0/legalcode) | |
Record name | 2-(4-(1-(Quinolin-6-ylmethyl)-1H-(1,2,3)triazolo(4,5-b)pyrazin-6-yl)-1H-pyrazol-1-yl)ethanol | |
Source | EPA DSSTox | |
URL | https://comptox.epa.gov/dashboard/DTXSID401026097 | |
Description | DSSTox provides a high quality public chemistry resource for supporting improved predictive toxicology. | |
Record name | PF-04217903 | |
Source | FDA Global Substance Registration System (GSRS) | |
URL | https://gsrs.ncats.nih.gov/ginas/app/beta/substances/CYJ9ATV1IJ | |
Description | The FDA Global Substance Registration System (GSRS) enables the efficient and accurate exchange of information on what substances are in regulated products. Instead of relying on names, which vary across regulatory domains, countries, and regions, the GSRS knowledge base makes it possible for substances to be defined by standardized, scientific descriptions. | |
Explanation | Unless otherwise noted, the contents of the FDA website (www.fda.gov), both text and graphics, are not copyrighted. They are in the public domain and may be republished, reprinted and otherwise used freely by anyone without the need to obtain permission from FDA. Credit to the U.S. Food and Drug Administration as the source is appreciated but not required. | |
Synthesis routes and methods I
Procedure details
Synthesis routes and methods II
Procedure details
Retrosynthesis Analysis
AI-Powered Synthesis Planning: Our tool employs the Template_relevance Pistachio, Template_relevance Bkms_metabolic, Template_relevance Pistachio_ringbreaker, Template_relevance Reaxys, Template_relevance Reaxys_biocatalysis model, leveraging a vast database of chemical reactions to predict feasible synthetic routes.
One-Step Synthesis Focus: Specifically designed for one-step synthesis, it provides concise and direct routes for your target compounds, streamlining the synthesis process.
Accurate Predictions: Utilizing the extensive PISTACHIO, BKMS_METABOLIC, PISTACHIO_RINGBREAKER, REAXYS, REAXYS_BIOCATALYSIS database, our tool offers high-accuracy predictions, reflecting the latest in chemical research and data.
Strategy Settings
Precursor scoring | Relevance Heuristic |
---|---|
Min. plausibility | 0.01 |
Model | Template_relevance |
Template Set | Pistachio/Bkms_metabolic/Pistachio_ringbreaker/Reaxys/Reaxys_biocatalysis |
Top-N result to add to graph | 6 |
Feasible Synthetic Routes
Disclaimer and Information on In-Vitro Research Products
Please be aware that all articles and product information presented on BenchChem are intended solely for informational purposes. The products available for purchase on BenchChem are specifically designed for in-vitro studies, which are conducted outside of living organisms. In-vitro studies, derived from the Latin term "in glass," involve experiments performed in controlled laboratory settings using cells or tissues. It is important to note that these products are not categorized as medicines or drugs, and they have not received approval from the FDA for the prevention, treatment, or cure of any medical condition, ailment, or disease. We must emphasize that any form of bodily introduction of these products into humans or animals is strictly prohibited by law. It is essential to adhere to these guidelines to ensure compliance with legal and ethical standards in research and experimentation.