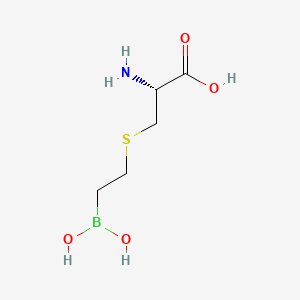
S-(2-boronoethyl)-L-cysteine
Overview
Description
Bose-Einstein Condensate (BEC) is a state of matter that occurs when a gas of bosons is cooled to temperatures very close to absolute zero (0 Kelvin or -273.15 degrees Celsius). Under these conditions, a large fraction of the bosons occupy the lowest quantum state, resulting in macroscopic quantum phenomena, such as wavefunction interference, becoming apparent .
Mechanism of Action
Target of Action
S-(2-boronoethyl)-L-cysteine is primarily used in Boron Neutron Capture Therapy (BNCT) . The primary targets of this compound are cancer cells, where it preferentially accumulates . This selective targeting is achieved through the use of peptide transporter 1 (PepT1), an oligopeptide transporter whose expression is increased in tumor cells .
Mode of Action
Once the compound enters the tumor cells, it interacts with its targets through a nuclear reaction. When irradiated by low-energy thermal neutrons, the boron-10 (^10B) isotope in the compound becomes unstable and decays, releasing high-energy alpha particles (^4He) and recoil lithium particles (^7Li) . This fission reaction releases a large amount of energy in a narrow region, causing intracellular double-strand DNA breaks leading to apoptosis .
Biochemical Pathways
The biochemical pathway involved in the action of this compound is the boron neutron capture reaction . This reaction is triggered when the boron atoms in the compound capture thermal neutrons, leading to the release of alpha particles and recoil lithium particles . The energy released from this reaction causes DNA damage in the cancer cells, leading to their death .
Pharmacokinetics
It is known that the compound is administered to patients through infusion . The compound then selectively accumulates in cancer cells, enhancing its bioavailability at the target site .
Result of Action
The result of the action of this compound is the selective killing of cancer cells . The high-energy particles released during the boron neutron capture reaction cause DNA damage in the cancer cells, leading to apoptosis . This selective action minimizes damage to surrounding healthy tissues .
Action Environment
The action of this compound is influenced by the local immune environment . For instance, the infiltration of macrophages and the up-regulation of alternatively activated macrophage markers are dependent on interleukin-4 (IL-4) or interleukin-13 (IL-13) activation . These factors can influence the compound’s action, efficacy, and stability .
Biochemical Analysis
Biochemical Properties
S-(2-boronoethyl)-L-cysteine is known to interact with arginase, an enzyme that plays a crucial role in the urea cycle, which is responsible for the detoxification of ammonia in the body . The compound acts as a reversible competitive inhibitor of arginase . This interaction with arginase suggests that this compound could potentially influence various biochemical reactions within the body.
Cellular Effects
The effects of this compound on cells are largely tied to its interaction with arginase. In a study involving mice infected with Nippostrongylus brasiliensis, this compound was administered in drinking water to inhibit arginase activity . The results showed that specific inhibition of arginase activity interfered with smooth muscle contractility . This suggests that this compound can influence cell function, potentially impacting cell signaling pathways and cellular metabolism.
Molecular Mechanism
The molecular mechanism of this compound is primarily related to its role as an arginase inhibitor. By binding to arginase, this compound prevents the enzyme from catalyzing the conversion of L-arginine to L-ornithine and urea . This inhibition can lead to changes in gene expression and can affect various biological processes at the molecular level.
Metabolic Pathways
This compound is involved in the urea cycle through its inhibition of arginase This could potentially affect metabolic flux and metabolite levels within the body
Preparation Methods
The preparation of Bose-Einstein Condensate involves cooling a dilute gas of bosons to extremely low temperatures using laser cooling and evaporative cooling techniques. The process typically involves the following steps:
Laser Cooling: Atoms are cooled using laser beams that slow down their motion.
Magnetic Trapping: The cooled atoms are trapped using magnetic fields.
Evaporative Cooling: The trapped atoms are further cooled by selectively removing the higher-energy atoms, allowing the remaining atoms to reach even lower temperatures
Chemical Reactions Analysis
Bose-Einstein Condensate itself does not undergo chemical reactions in the traditional sense, as it is a state of matter rather than a chemical compound. it can exhibit unique quantum phenomena such as superfluidity and macroscopic quantum interference. These phenomena are studied using various experimental techniques, including spectroscopy and interferometry .
Scientific Research Applications
Bose-Einstein Condensate has numerous scientific research applications, including:
Quantum Simulation: BECs are used to simulate complex quantum systems and study quantum many-body phenomena.
Atom Lasers: BECs can be used to create coherent beams of atoms, similar to how lasers create coherent beams of light.
Precision Measurements: BECs are used in atomic clocks and sensors for measuring gravitational, rotational, and magnetic fields with high precision.
Fundamental Physics: BECs enable the study of fundamental quantum mechanics, including tests of quantum superposition and entanglement .
Comparison with Similar Compounds
Bose-Einstein Condensate is unique among states of matter due to its macroscopic quantum properties. Similar states of matter include:
Fermionic Condensate: A state of matter formed by fermions at low temperatures, exhibiting superfluidity.
Superfluid Helium: Helium-4 and Helium-3 can form superfluid phases at low temperatures, exhibiting properties similar to BEC.
Superconductors: Materials that exhibit zero electrical resistance and expulsion of magnetic fields below a critical temperature, described by Bose-Einstein condensation of Cooper pairs .
Bose-Einstein Condensate is unique in that it involves the macroscopic occupation of the lowest quantum state by bosons, leading to phenomena that are not observed in other states of matter.
Properties
IUPAC Name |
(2R)-2-amino-3-(2-boronoethylsulfanyl)propanoic acid | |
---|---|---|
Source | PubChem | |
URL | https://pubchem.ncbi.nlm.nih.gov | |
Description | Data deposited in or computed by PubChem | |
InChI |
InChI=1S/C5H12BNO4S/c7-4(5(8)9)3-12-2-1-6(10)11/h4,10-11H,1-3,7H2,(H,8,9)/t4-/m0/s1 | |
Source | PubChem | |
URL | https://pubchem.ncbi.nlm.nih.gov | |
Description | Data deposited in or computed by PubChem | |
InChI Key |
OTJHLDXXJHAZTN-BYPYZUCNSA-N | |
Source | PubChem | |
URL | https://pubchem.ncbi.nlm.nih.gov | |
Description | Data deposited in or computed by PubChem | |
Canonical SMILES |
B(CCSCC(C(=O)O)N)(O)O | |
Source | PubChem | |
URL | https://pubchem.ncbi.nlm.nih.gov | |
Description | Data deposited in or computed by PubChem | |
Isomeric SMILES |
B(CCSC[C@@H](C(=O)O)N)(O)O | |
Source | PubChem | |
URL | https://pubchem.ncbi.nlm.nih.gov | |
Description | Data deposited in or computed by PubChem | |
Molecular Formula |
C5H12BNO4S | |
Source | PubChem | |
URL | https://pubchem.ncbi.nlm.nih.gov | |
Description | Data deposited in or computed by PubChem | |
DSSTOX Substance ID |
DTXSID00432180 | |
Record name | S-(2-boronoethyl)-L-cysteine | |
Source | EPA DSSTox | |
URL | https://comptox.epa.gov/dashboard/DTXSID00432180 | |
Description | DSSTox provides a high quality public chemistry resource for supporting improved predictive toxicology. | |
Molecular Weight |
193.03 g/mol | |
Source | PubChem | |
URL | https://pubchem.ncbi.nlm.nih.gov | |
Description | Data deposited in or computed by PubChem | |
CAS No. |
63107-40-4 | |
Record name | S-(2-boronoethyl)-L-cysteine | |
Source | EPA DSSTox | |
URL | https://comptox.epa.gov/dashboard/DTXSID00432180 | |
Description | DSSTox provides a high quality public chemistry resource for supporting improved predictive toxicology. | |
Retrosynthesis Analysis
AI-Powered Synthesis Planning: Our tool employs the Template_relevance Pistachio, Template_relevance Bkms_metabolic, Template_relevance Pistachio_ringbreaker, Template_relevance Reaxys, Template_relevance Reaxys_biocatalysis model, leveraging a vast database of chemical reactions to predict feasible synthetic routes.
One-Step Synthesis Focus: Specifically designed for one-step synthesis, it provides concise and direct routes for your target compounds, streamlining the synthesis process.
Accurate Predictions: Utilizing the extensive PISTACHIO, BKMS_METABOLIC, PISTACHIO_RINGBREAKER, REAXYS, REAXYS_BIOCATALYSIS database, our tool offers high-accuracy predictions, reflecting the latest in chemical research and data.
Strategy Settings
Precursor scoring | Relevance Heuristic |
---|---|
Min. plausibility | 0.01 |
Model | Template_relevance |
Template Set | Pistachio/Bkms_metabolic/Pistachio_ringbreaker/Reaxys/Reaxys_biocatalysis |
Top-N result to add to graph | 6 |
Feasible Synthetic Routes
Q1: How does S-(2-boronoethyl)-L-cysteine (BEC) interact with arginase?
A1: BEC acts as a transition-state analog inhibitor of arginase. [, ] Its boronic acid moiety forms a tetrahedral boronate anion by nucleophilic attack from the metal-bridging hydroxide ion within the arginase active site. This boronate then bridges the binuclear manganese cluster of arginase, mimicking the transition state of L-arginine hydrolysis, thus inhibiting the enzyme. []
Q2: What are the downstream effects of arginase inhibition by BEC?
A2: Inhibiting arginase with BEC increases the availability of L-arginine for NOS. [, ] This leads to enhanced NO production, impacting various physiological processes like vasodilation, [, , , ] cell proliferation, [, ] and immune response. [, ]
Q3: Does BEC affect both arginase isoforms (arginase I and arginase II) equally?
A3: While BEC inhibits both arginase I and arginase II, research suggests a more pronounced role of arginase II in specific pathologies like diabetic nephropathy. [, ] Studies using arginase II-deficient mice or selective inhibition strategies highlight the significance of targeting arginase II for therapeutic benefits. [, ]
Q4: What is the molecular formula and weight of BEC?
A4: The molecular formula of BEC is C5H12BN2O4S, and its molecular weight is 206.03 g/mol.
Q5: Is there information available about the material compatibility and stability of BEC under various conditions?
A5: The provided research papers primarily focus on the biological activity and therapeutic potential of BEC. Information regarding its material compatibility and stability under various conditions is limited in these studies.
Q6: Does BEC possess any catalytic properties itself?
A6: BEC primarily functions as an enzyme inhibitor and does not exhibit intrinsic catalytic properties. Its primary mode of action involves binding to arginase and preventing its catalytic activity.
Q7: Have computational studies been conducted on BEC and its interactions with arginase?
A7: Yes, computational chemistry, including molecular modeling and docking studies, has been employed to investigate the binding mode of BEC to the arginase active site. [, , ] These studies provide insights into the structural basis of its high affinity and selectivity for arginase.
Q8: How do modifications to the structure of BEC affect its activity and selectivity?
A8: Research indicates that the distance between the boronic acid group and the α-amino acid function in BEC analogs is crucial for arginase inhibition. [] Additionally, the position of the sulfur atom in the side chain influences its interaction with the arginase active site. [] Modifications altering these structural features can significantly impact BEC's inhibitory potency and selectivity.
Q9: What is known about the stability of BEC and strategies to enhance its formulation?
A9: The provided research papers primarily focus on BEC's in vitro and in vivo biological effects. Detailed investigations on its stability under various conditions and specific formulation approaches to improve its stability, solubility, or bioavailability are not extensively covered.
Q10: What are the SHE regulations surrounding the use of BEC?
A10: The provided research focuses on the scientific understanding of BEC. Information on specific SHE regulations and compliance requirements falls outside the scope of these studies. Always consult relevant safety data sheets and regulatory guidelines when handling and using any chemical substance.
Q11: What is the pharmacokinetic profile of BEC?
A11: Details on the absorption, distribution, metabolism, and excretion (ADME) of BEC are not extensively discussed within the provided research papers.
Q12: Has BEC demonstrated efficacy in preclinical models of disease?
A12: Yes, BEC has shown promising results in various preclinical disease models. For instance, it attenuated neointima formation in rat carotid artery injury models, [, ] reduced pulmonary artery hypertension in rats, [] and improved endothelial dysfunction in diabetic rat models. [, , ]
Disclaimer and Information on In-Vitro Research Products
Please be aware that all articles and product information presented on BenchChem are intended solely for informational purposes. The products available for purchase on BenchChem are specifically designed for in-vitro studies, which are conducted outside of living organisms. In-vitro studies, derived from the Latin term "in glass," involve experiments performed in controlled laboratory settings using cells or tissues. It is important to note that these products are not categorized as medicines or drugs, and they have not received approval from the FDA for the prevention, treatment, or cure of any medical condition, ailment, or disease. We must emphasize that any form of bodily introduction of these products into humans or animals is strictly prohibited by law. It is essential to adhere to these guidelines to ensure compliance with legal and ethical standards in research and experimentation.