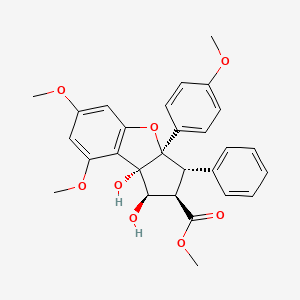
Aglafoline
Overview
Description
Aglafolin is a heterotricyclic compound based on a 2,3,3a,8b-tetrahydro-1H-benzo[b]cyclopenta[d]furan framework. It is substituted by hydroxy groups at positions C-1 and C-8b, a methoxycarbonyl group at C-2, a phenyl group at C-3, a 4-methoxyphenyl group at C-3a, and methoxy groups at C-6 and C-8 . Aglafolin is known for its role as a platelet aggregation inhibitor and is found in plants such as Aglaia elliptifolia and Aglaia odorata .
Preparation Methods
The synthesis of Aglafolin involves several steps, typically starting with the extraction from natural sources like Aglaia elliptifolia. The compound can also be synthesized through a series of chemical reactions involving the formation of the benzo[b]cyclopenta[d]furan framework and subsequent substitutions at specific positions .
Chemical Reactions Analysis
Aglafolin undergoes various chemical reactions, including:
Oxidation: Aglafolin can be oxidized to form different derivatives, depending on the reagents and conditions used.
Reduction: The compound can be reduced to alter its functional groups, leading to different products.
Substitution: Aglafolin can undergo substitution reactions, particularly at the methoxy and hydroxy groups.
Common reagents used in these reactions include oxidizing agents like potassium permanganate and reducing agents like sodium borohydride. The major products formed from these reactions depend on the specific conditions and reagents used .
Scientific Research Applications
Aglafolin has a wide range of scientific research applications:
Chemistry: Used as a model compound for studying heterotricyclic frameworks and their reactivity.
Industry: Potential applications in the development of new pharmaceuticals and therapeutic agents.
Mechanism of Action
Aglafolin exerts its effects primarily by inhibiting platelet aggregation. It antagonizes the platelet-activating factor (PAF), preventing the aggregation and ATP release reaction in platelets . The molecular targets include PAF receptors on the platelet surface, and the pathways involved are related to the inhibition of thromboxane B2 formation and inositol monophosphate formation .
Comparison with Similar Compounds
Aglafolin is similar to other compounds like rocaglamide, which also has a heterotricyclic framework and exhibits cytotoxic and anti-platelet aggregation properties . Aglafolin is unique in its specific substitutions and the precise positions of its functional groups, which contribute to its distinct biological activities .
Similar Compounds
- Rocaglamide
- Methyl rocaglate
- Other derivatives of the benzo[b]cyclopenta[d]furan framework .
Aglafolin’s unique structure and biological activities make it a compound of significant interest in various fields of scientific research.
Properties
IUPAC Name |
methyl (1R,2R,3S,3aR,8bS)-1,8b-dihydroxy-6,8-dimethoxy-3a-(4-methoxyphenyl)-3-phenyl-2,3-dihydro-1H-cyclopenta[b][1]benzofuran-2-carboxylate | |
---|---|---|
Source | PubChem | |
URL | https://pubchem.ncbi.nlm.nih.gov | |
Description | Data deposited in or computed by PubChem | |
InChI |
InChI=1S/C28H28O8/c1-32-18-12-10-17(11-13-18)28-23(16-8-6-5-7-9-16)22(26(30)35-4)25(29)27(28,31)24-20(34-3)14-19(33-2)15-21(24)36-28/h5-15,22-23,25,29,31H,1-4H3/t22-,23-,25-,27+,28+/m1/s1 | |
Source | PubChem | |
URL | https://pubchem.ncbi.nlm.nih.gov | |
Description | Data deposited in or computed by PubChem | |
InChI Key |
VFTGDXPPYSWBSO-GWNOIRNCSA-N | |
Source | PubChem | |
URL | https://pubchem.ncbi.nlm.nih.gov | |
Description | Data deposited in or computed by PubChem | |
Canonical SMILES |
COC1=CC=C(C=C1)C23C(C(C(C2(C4=C(O3)C=C(C=C4OC)OC)O)O)C(=O)OC)C5=CC=CC=C5 | |
Source | PubChem | |
URL | https://pubchem.ncbi.nlm.nih.gov | |
Description | Data deposited in or computed by PubChem | |
Isomeric SMILES |
COC1=CC=C(C=C1)[C@]23[C@@H]([C@H]([C@H]([C@]2(C4=C(O3)C=C(C=C4OC)OC)O)O)C(=O)OC)C5=CC=CC=C5 | |
Source | PubChem | |
URL | https://pubchem.ncbi.nlm.nih.gov | |
Description | Data deposited in or computed by PubChem | |
Molecular Formula |
C28H28O8 | |
Source | PubChem | |
URL | https://pubchem.ncbi.nlm.nih.gov | |
Description | Data deposited in or computed by PubChem | |
DSSTOX Substance ID |
DTXSID50932140 | |
Record name | Methyl 1,8b-dihydroxy-6,8-dimethoxy-3a-(4-methoxyphenyl)-3-phenyl-2,3,3a,8b-tetrahydro-1H-benzo[b]cyclopenta[d]furan-2-carboxylate | |
Source | EPA DSSTox | |
URL | https://comptox.epa.gov/dashboard/DTXSID50932140 | |
Description | DSSTox provides a high quality public chemistry resource for supporting improved predictive toxicology. | |
Molecular Weight |
492.5 g/mol | |
Source | PubChem | |
URL | https://pubchem.ncbi.nlm.nih.gov | |
Description | Data deposited in or computed by PubChem | |
CAS No. |
143901-35-3 | |
Record name | Methyl (1R,2R,3S,3aR,8bS)-2,3,3a,8b-tetrahydro-1,8b-dihydroxy-6,8-dimethoxy-3a-(4-methoxyphenyl)-3-phenyl-1H-cyclopenta[b]benzofuran-2-carboxylate | |
Source | CAS Common Chemistry | |
URL | https://commonchemistry.cas.org/detail?cas_rn=143901-35-3 | |
Description | CAS Common Chemistry is an open community resource for accessing chemical information. Nearly 500,000 chemical substances from CAS REGISTRY cover areas of community interest, including common and frequently regulated chemicals, and those relevant to high school and undergraduate chemistry classes. This chemical information, curated by our expert scientists, is provided in alignment with our mission as a division of the American Chemical Society. | |
Explanation | The data from CAS Common Chemistry is provided under a CC-BY-NC 4.0 license, unless otherwise stated. | |
Record name | Aglafoline | |
Source | ChemIDplus | |
URL | https://pubchem.ncbi.nlm.nih.gov/substance/?source=chemidplus&sourceid=0143901353 | |
Description | ChemIDplus is a free, web search system that provides access to the structure and nomenclature authority files used for the identification of chemical substances cited in National Library of Medicine (NLM) databases, including the TOXNET system. | |
Record name | Methyl 1,8b-dihydroxy-6,8-dimethoxy-3a-(4-methoxyphenyl)-3-phenyl-2,3,3a,8b-tetrahydro-1H-benzo[b]cyclopenta[d]furan-2-carboxylate | |
Source | EPA DSSTox | |
URL | https://comptox.epa.gov/dashboard/DTXSID50932140 | |
Description | DSSTox provides a high quality public chemistry resource for supporting improved predictive toxicology. | |
Retrosynthesis Analysis
AI-Powered Synthesis Planning: Our tool employs the Template_relevance Pistachio, Template_relevance Bkms_metabolic, Template_relevance Pistachio_ringbreaker, Template_relevance Reaxys, Template_relevance Reaxys_biocatalysis model, leveraging a vast database of chemical reactions to predict feasible synthetic routes.
One-Step Synthesis Focus: Specifically designed for one-step synthesis, it provides concise and direct routes for your target compounds, streamlining the synthesis process.
Accurate Predictions: Utilizing the extensive PISTACHIO, BKMS_METABOLIC, PISTACHIO_RINGBREAKER, REAXYS, REAXYS_BIOCATALYSIS database, our tool offers high-accuracy predictions, reflecting the latest in chemical research and data.
Strategy Settings
Precursor scoring | Relevance Heuristic |
---|---|
Min. plausibility | 0.01 |
Model | Template_relevance |
Template Set | Pistachio/Bkms_metabolic/Pistachio_ringbreaker/Reaxys/Reaxys_biocatalysis |
Top-N result to add to graph | 6 |
Feasible Synthetic Routes
Disclaimer and Information on In-Vitro Research Products
Please be aware that all articles and product information presented on BenchChem are intended solely for informational purposes. The products available for purchase on BenchChem are specifically designed for in-vitro studies, which are conducted outside of living organisms. In-vitro studies, derived from the Latin term "in glass," involve experiments performed in controlled laboratory settings using cells or tissues. It is important to note that these products are not categorized as medicines or drugs, and they have not received approval from the FDA for the prevention, treatment, or cure of any medical condition, ailment, or disease. We must emphasize that any form of bodily introduction of these products into humans or animals is strictly prohibited by law. It is essential to adhere to these guidelines to ensure compliance with legal and ethical standards in research and experimentation.