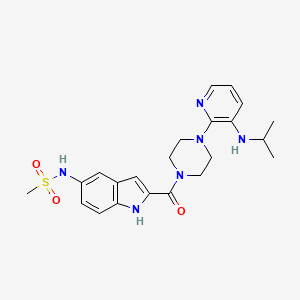
Delavirdine
Overview
Description
Delavirdine is a non-nucleoside reverse transcriptase inhibitor used primarily in the treatment of Human Immunodeficiency Virus (HIV) type 1 infections. It is known for its ability to bind directly to the reverse transcriptase enzyme, thereby inhibiting the replication of the virus. This compound is marketed under the brand name Rescriptor and is typically used as part of highly active antiretroviral therapy (HAART) for managing HIV infections .
Mechanism of Action
Target of Action
Delavirdine primarily targets the reverse transcriptase (RT) enzyme of the Human Immunodeficiency Virus Type 1 (HIV-1) . This enzyme plays a crucial role in the replication of the virus, making it a key target for antiretroviral therapy .
Mode of Action
This compound is a non-nucleoside reverse transcriptase inhibitor (nNRTI). It binds directly to the RT enzyme, causing a disruption of the enzyme’s catalytic site . This action blocks both the RNA-dependent and DNA-dependent DNA polymerase activities of the enzyme .
Biochemical Pathways
By inhibiting the RT enzyme, this compound prevents the conversion of viral RNA into DNA, a critical step in the viral replication process . This disruption of the viral life cycle prevents the proliferation of the virus and reduces the viral load in the body .
Pharmacokinetics
This compound exhibits nonlinear steady-state elimination pharmacokinetics . It is rapidly absorbed after oral administration . The absorption of this compound is reduced by gastric hypoacidity . It is bound extensively to plasma proteins, primarily albumin . This compound undergoes extensive metabolism in the liver via cytochrome P450 (CYP), with little urinary excretion of unchanged drug . The major metabolic pathway of this compound is N-dealkylation, which is mediated by CYP3A .
Result of Action
The inhibition of the RT enzyme by this compound results in a decrease in the replication of HIV-1 . This leads to a reduction in the viral load and an increase in CD4 cell counts, improving the immune response against the virus .
Action Environment
The action of this compound can be influenced by various environmental factors. For instance, the absorption of this compound is reduced in conditions of gastric hypoacidity . Additionally, certain drugs that induce or inhibit CYP enzymes can affect the metabolism and efficacy of this compound .
Biochemical Analysis
Biochemical Properties
Delavirdine binds directly to reverse transcriptase (RT) and blocks the RNA-dependent and DNA-dependent DNA polymerase activities by causing a disruption of the enzyme’s catalytic site . The activity of this compound does not compete with template or nucleoside triphosphates .
Cellular Effects
This compound, as an inhibitor of HIV-1 reverse transcriptase, plays a crucial role in preventing the replication of the HIV-1 virus in cells. By blocking the function of reverse transcriptase, this compound prevents the virus from creating more copies of itself, thereby reducing viral load and slowing the progression of the disease .
Molecular Mechanism
This compound exerts its effects at the molecular level by binding directly to the reverse transcriptase enzyme. This binding disrupts the enzyme’s catalytic site, blocking both RNA-dependent and DNA-dependent DNA polymerase activities . This prevents the virus from replicating its genetic material and producing new virus particles .
Temporal Effects in Laboratory Settings
As of now, it is known that this compound is a potent and specific inhibitor of HIV-1 reverse transcriptase .
Dosage Effects in Animal Models
It is known that this compound is used in combination with other antiretroviral agents for the treatment of HIV-1 infection .
Metabolic Pathways
This compound undergoes extensive metabolism by cytochrome P450 (CYP) with little urinary excretion of unchanged drug .
Transport and Distribution
The transport and distribution of this compound within cells and tissues are complex processes that involve various transporters and binding proteins. This compound is known to inhibit the CYP3A4-mediated metabolism of HIV protease inhibitors, thereby increasing systemic exposure to protease inhibitors .
Subcellular Localization
As an inhibitor of reverse transcriptase, this compound is expected to localize where the HIV-1 virus replicates, which is within the host cell’s cytoplasm .
Preparation Methods
Synthetic Routes and Reaction Conditions: Delavirdine is synthesized through a multi-step process involving the formation of key intermediates. The synthesis begins with the preparation of 5-methylsulfonamidoindole-2-carboxylic acid, which is then converted into its imidazolide derivative. This intermediate undergoes acylation with a piperazine derivative to form the final product .
Industrial Production Methods: Industrial production of this compound involves optimizing the synthetic route to ensure high yield and purity. The process typically includes steps such as reductive alkylation, acylation, and purification through crystallization or chromatography .
Chemical Reactions Analysis
Types of Reactions: Delavirdine undergoes various chemical reactions, including:
Oxidation: this compound can be oxidized to form sulfoxide and sulfone derivatives.
Reduction: Reduction reactions can convert this compound into its corresponding amine derivatives.
Substitution: Nucleophilic substitution reactions can modify the piperazine ring or the indole moiety.
Common Reagents and Conditions:
Oxidation: Common oxidizing agents include hydrogen peroxide and m-chloroperbenzoic acid.
Reduction: Reducing agents such as lithium aluminum hydride or sodium borohydride are used.
Substitution: Nucleophiles like amines or thiols can be used under basic conditions.
Major Products Formed:
Oxidation: Sulfoxide and sulfone derivatives.
Reduction: Amine derivatives.
Substitution: Modified piperazine or indole derivatives.
Scientific Research Applications
Delavirdine has a wide range of applications in scientific research, including:
Chemistry: Used as a model compound for studying non-nucleoside reverse transcriptase inhibitors.
Biology: Investigated for its effects on viral replication and resistance mechanisms.
Medicine: Employed in clinical trials for optimizing HIV treatment regimens.
Industry: Utilized in the development of new antiretroviral drugs and formulations
Comparison with Similar Compounds
Efavirenz: Another non-nucleoside reverse transcriptase inhibitor with a higher efficacy but more side effects.
Nevirapine: Known for its use in preventing mother-to-child transmission of HIV.
Etravirine: A second-generation non-nucleoside reverse transcriptase inhibitor with a broader resistance profile
Uniqueness of Delavirdine: this compound is unique due to its specific binding affinity for the reverse transcriptase enzyme and its ability to increase the plasma concentration of several protease inhibitors. its use is limited by its lower efficacy compared to other non-nucleoside reverse transcriptase inhibitors and its complex drug interaction profile .
Properties
IUPAC Name |
N-[2-[4-[3-(propan-2-ylamino)pyridin-2-yl]piperazine-1-carbonyl]-1H-indol-5-yl]methanesulfonamide | |
---|---|---|
Source | PubChem | |
URL | https://pubchem.ncbi.nlm.nih.gov | |
Description | Data deposited in or computed by PubChem | |
InChI |
InChI=1S/C22H28N6O3S/c1-15(2)24-19-5-4-8-23-21(19)27-9-11-28(12-10-27)22(29)20-14-16-13-17(26-32(3,30)31)6-7-18(16)25-20/h4-8,13-15,24-26H,9-12H2,1-3H3 | |
Source | PubChem | |
URL | https://pubchem.ncbi.nlm.nih.gov | |
Description | Data deposited in or computed by PubChem | |
InChI Key |
WHBIGIKBNXZKFE-UHFFFAOYSA-N | |
Source | PubChem | |
URL | https://pubchem.ncbi.nlm.nih.gov | |
Description | Data deposited in or computed by PubChem | |
Canonical SMILES |
CC(C)NC1=C(N=CC=C1)N2CCN(CC2)C(=O)C3=CC4=C(N3)C=CC(=C4)NS(=O)(=O)C | |
Source | PubChem | |
URL | https://pubchem.ncbi.nlm.nih.gov | |
Description | Data deposited in or computed by PubChem | |
Molecular Formula |
C22H28N6O3S | |
Source | PubChem | |
URL | https://pubchem.ncbi.nlm.nih.gov | |
Description | Data deposited in or computed by PubChem | |
Related CAS |
147221-93-0 (mesylate) | |
Record name | Delavirdine [INN] | |
Source | ChemIDplus | |
URL | https://pubchem.ncbi.nlm.nih.gov/substance/?source=chemidplus&sourceid=0136817599 | |
Description | ChemIDplus is a free, web search system that provides access to the structure and nomenclature authority files used for the identification of chemical substances cited in National Library of Medicine (NLM) databases, including the TOXNET system. | |
DSSTOX Substance ID |
DTXSID6022892 | |
Record name | Delavirdine | |
Source | EPA DSSTox | |
URL | https://comptox.epa.gov/dashboard/DTXSID6022892 | |
Description | DSSTox provides a high quality public chemistry resource for supporting improved predictive toxicology. | |
Molecular Weight |
456.6 g/mol | |
Source | PubChem | |
URL | https://pubchem.ncbi.nlm.nih.gov | |
Description | Data deposited in or computed by PubChem | |
Physical Description |
Solid | |
Record name | Delavirdine | |
Source | Human Metabolome Database (HMDB) | |
URL | http://www.hmdb.ca/metabolites/HMDB0014843 | |
Description | The Human Metabolome Database (HMDB) is a freely available electronic database containing detailed information about small molecule metabolites found in the human body. | |
Explanation | HMDB is offered to the public as a freely available resource. Use and re-distribution of the data, in whole or in part, for commercial purposes requires explicit permission of the authors and explicit acknowledgment of the source material (HMDB) and the original publication (see the HMDB citing page). We ask that users who download significant portions of the database cite the HMDB paper in any resulting publications. | |
Solubility |
8.60e-02 g/L | |
Record name | Delavirdine | |
Source | Human Metabolome Database (HMDB) | |
URL | http://www.hmdb.ca/metabolites/HMDB0014843 | |
Description | The Human Metabolome Database (HMDB) is a freely available electronic database containing detailed information about small molecule metabolites found in the human body. | |
Explanation | HMDB is offered to the public as a freely available resource. Use and re-distribution of the data, in whole or in part, for commercial purposes requires explicit permission of the authors and explicit acknowledgment of the source material (HMDB) and the original publication (see the HMDB citing page). We ask that users who download significant portions of the database cite the HMDB paper in any resulting publications. | |
Mechanism of Action |
Delavirdine binds directly to viral reverse transcriptase (RT) and blocks the RNA-dependent and DNA-dependent DNA polymerase activities by disrupting the enzyme's catalytic site. | |
Record name | Delavirdine | |
Source | DrugBank | |
URL | https://www.drugbank.ca/drugs/DB00705 | |
Description | The DrugBank database is a unique bioinformatics and cheminformatics resource that combines detailed drug (i.e. chemical, pharmacological and pharmaceutical) data with comprehensive drug target (i.e. sequence, structure, and pathway) information. | |
Explanation | Creative Common's Attribution-NonCommercial 4.0 International License (http://creativecommons.org/licenses/by-nc/4.0/legalcode) | |
CAS No. |
136817-59-9 | |
Record name | Delavirdine | |
Source | CAS Common Chemistry | |
URL | https://commonchemistry.cas.org/detail?cas_rn=136817-59-9 | |
Description | CAS Common Chemistry is an open community resource for accessing chemical information. Nearly 500,000 chemical substances from CAS REGISTRY cover areas of community interest, including common and frequently regulated chemicals, and those relevant to high school and undergraduate chemistry classes. This chemical information, curated by our expert scientists, is provided in alignment with our mission as a division of the American Chemical Society. | |
Explanation | The data from CAS Common Chemistry is provided under a CC-BY-NC 4.0 license, unless otherwise stated. | |
Record name | Delavirdine [INN] | |
Source | ChemIDplus | |
URL | https://pubchem.ncbi.nlm.nih.gov/substance/?source=chemidplus&sourceid=0136817599 | |
Description | ChemIDplus is a free, web search system that provides access to the structure and nomenclature authority files used for the identification of chemical substances cited in National Library of Medicine (NLM) databases, including the TOXNET system. | |
Record name | Delavirdine | |
Source | DrugBank | |
URL | https://www.drugbank.ca/drugs/DB00705 | |
Description | The DrugBank database is a unique bioinformatics and cheminformatics resource that combines detailed drug (i.e. chemical, pharmacological and pharmaceutical) data with comprehensive drug target (i.e. sequence, structure, and pathway) information. | |
Explanation | Creative Common's Attribution-NonCommercial 4.0 International License (http://creativecommons.org/licenses/by-nc/4.0/legalcode) | |
Record name | Delavirdine | |
Source | EPA DSSTox | |
URL | https://comptox.epa.gov/dashboard/DTXSID6022892 | |
Description | DSSTox provides a high quality public chemistry resource for supporting improved predictive toxicology. | |
Record name | DELAVIRDINE | |
Source | FDA Global Substance Registration System (GSRS) | |
URL | https://gsrs.ncats.nih.gov/ginas/app/beta/substances/DOL5F9JD3E | |
Description | The FDA Global Substance Registration System (GSRS) enables the efficient and accurate exchange of information on what substances are in regulated products. Instead of relying on names, which vary across regulatory domains, countries, and regions, the GSRS knowledge base makes it possible for substances to be defined by standardized, scientific descriptions. | |
Explanation | Unless otherwise noted, the contents of the FDA website (www.fda.gov), both text and graphics, are not copyrighted. They are in the public domain and may be republished, reprinted and otherwise used freely by anyone without the need to obtain permission from FDA. Credit to the U.S. Food and Drug Administration as the source is appreciated but not required. | |
Record name | Delavirdine | |
Source | Human Metabolome Database (HMDB) | |
URL | http://www.hmdb.ca/metabolites/HMDB0014843 | |
Description | The Human Metabolome Database (HMDB) is a freely available electronic database containing detailed information about small molecule metabolites found in the human body. | |
Explanation | HMDB is offered to the public as a freely available resource. Use and re-distribution of the data, in whole or in part, for commercial purposes requires explicit permission of the authors and explicit acknowledgment of the source material (HMDB) and the original publication (see the HMDB citing page). We ask that users who download significant portions of the database cite the HMDB paper in any resulting publications. | |
Melting Point |
226-228 °C, 226 - 228 °C | |
Record name | Delavirdine | |
Source | DrugBank | |
URL | https://www.drugbank.ca/drugs/DB00705 | |
Description | The DrugBank database is a unique bioinformatics and cheminformatics resource that combines detailed drug (i.e. chemical, pharmacological and pharmaceutical) data with comprehensive drug target (i.e. sequence, structure, and pathway) information. | |
Explanation | Creative Common's Attribution-NonCommercial 4.0 International License (http://creativecommons.org/licenses/by-nc/4.0/legalcode) | |
Record name | Delavirdine | |
Source | Human Metabolome Database (HMDB) | |
URL | http://www.hmdb.ca/metabolites/HMDB0014843 | |
Description | The Human Metabolome Database (HMDB) is a freely available electronic database containing detailed information about small molecule metabolites found in the human body. | |
Explanation | HMDB is offered to the public as a freely available resource. Use and re-distribution of the data, in whole or in part, for commercial purposes requires explicit permission of the authors and explicit acknowledgment of the source material (HMDB) and the original publication (see the HMDB citing page). We ask that users who download significant portions of the database cite the HMDB paper in any resulting publications. | |
Retrosynthesis Analysis
AI-Powered Synthesis Planning: Our tool employs the Template_relevance Pistachio, Template_relevance Bkms_metabolic, Template_relevance Pistachio_ringbreaker, Template_relevance Reaxys, Template_relevance Reaxys_biocatalysis model, leveraging a vast database of chemical reactions to predict feasible synthetic routes.
One-Step Synthesis Focus: Specifically designed for one-step synthesis, it provides concise and direct routes for your target compounds, streamlining the synthesis process.
Accurate Predictions: Utilizing the extensive PISTACHIO, BKMS_METABOLIC, PISTACHIO_RINGBREAKER, REAXYS, REAXYS_BIOCATALYSIS database, our tool offers high-accuracy predictions, reflecting the latest in chemical research and data.
Strategy Settings
Precursor scoring | Relevance Heuristic |
---|---|
Min. plausibility | 0.01 |
Model | Template_relevance |
Template Set | Pistachio/Bkms_metabolic/Pistachio_ringbreaker/Reaxys/Reaxys_biocatalysis |
Top-N result to add to graph | 6 |
Feasible Synthetic Routes
Q1: How does delavirdine interact with HIV-1 reverse transcriptase?
A: this compound acts as a non-competitive inhibitor of HIV-1 reverse transcriptase. It binds to a specific allosteric site on the enzyme, distinct from the active site where nucleoside analogues bind. This binding induces conformational changes that inhibit the enzyme's polymerase activity, preventing the conversion of viral RNA to proviral DNA. []
Q2: What are the downstream effects of this compound's inhibition of HIV-1 reverse transcriptase?
A: By inhibiting HIV-1 reverse transcriptase, this compound disrupts the viral replication cycle. This leads to a decrease in the production of new viral particles and ultimately helps to reduce viral load in infected individuals. [, ]
Q3: What is the molecular formula and weight of this compound?
A: this compound's molecular formula is C22H28N6O3S, and its molecular weight is 452.57 g/mol. [, ]
Q4: Is there any available spectroscopic data for this compound?
A: Yes, spectroscopic data, including 1H NMR, 13C NMR, and mass spectrometry (MS), have been used to characterize this compound and its metabolites. This information is essential for structural elucidation and analysis of the drug's metabolism. [, ]
Q5: Which cytochrome P450 (CYP) enzymes are primarily involved in this compound metabolism?
A: this compound is primarily metabolized by CYP3A4 and, to a lesser extent, by CYP2D6. [, ]
Q6: How does this compound interact with other drugs metabolized by CYP3A4?
A: this compound can inhibit the activity of CYP3A4. Co-administration with other drugs metabolized by this enzyme, such as protease inhibitors, may lead to increased plasma concentrations of these drugs, potentially enhancing their therapeutic effects or increasing the risk of adverse reactions. [, , , ]
Q7: Are there specific drug interactions with this compound that clinicians should be aware of?
A: Yes, co-administration of this compound with drugs that are substrates, inhibitors, or inducers of CYP3A4 can lead to clinically significant drug interactions. For instance, this compound increases systemic exposure to ritonavir when co-administered. [, ]
Q8: What are the known mechanisms of resistance to this compound?
A: Resistance to this compound primarily arises from mutations in the HIV-1 reverse transcriptase gene. A single point mutation at codon 236 (P236L) is a common mechanism of resistance, leading to decreased susceptibility to this compound. [, ]
Q9: Does cross-resistance exist between this compound and other NNRTIs?
A: Yes, cross-resistance has been observed between this compound and other NNRTIs, such as nevirapine and efavirenz. This is due to the shared binding site on the HIV-1 reverse transcriptase enzyme. [, , ]
Q10: What are the primary routes of this compound elimination?
A: this compound is primarily eliminated through metabolism, with feces being the major route of excretion. []
Q11: Does this compound penetrate the central nervous system (CNS)?
A: this compound demonstrates a brain/plasma pharmacokinetic disequilibrium, indicating limited CNS penetration. The cerebrospinal fluid concentration, a surrogate for unbound brain concentration, is approximately 5-fold lower than the corresponding unbound plasma concentration in humans. []
Q12: Has this compound demonstrated synergistic antiviral activity with other antiretroviral agents in vitro?
A: Yes, in vitro studies have shown that combining this compound with protease inhibitors like U-75875 or interferon-alpha can result in synergistic inhibition of HIV-1 replication. []
Q13: Has the efficacy of this compound been evaluated in clinical trials?
A: Yes, numerous clinical trials have assessed the efficacy and safety of this compound in combination with other antiretroviral agents. These trials have demonstrated that this compound-containing regimens can effectively suppress HIV-1 replication and increase CD4+ cell counts in HIV-infected individuals. [, , ]
Q14: What are the most common side effects associated with this compound therapy?
A: The most common side effect of this compound is skin rash, which can be severe in some cases. Other reported side effects include nausea, headache, and fatigue. [, ]
Disclaimer and Information on In-Vitro Research Products
Please be aware that all articles and product information presented on BenchChem are intended solely for informational purposes. The products available for purchase on BenchChem are specifically designed for in-vitro studies, which are conducted outside of living organisms. In-vitro studies, derived from the Latin term "in glass," involve experiments performed in controlled laboratory settings using cells or tissues. It is important to note that these products are not categorized as medicines or drugs, and they have not received approval from the FDA for the prevention, treatment, or cure of any medical condition, ailment, or disease. We must emphasize that any form of bodily introduction of these products into humans or animals is strictly prohibited by law. It is essential to adhere to these guidelines to ensure compliance with legal and ethical standards in research and experimentation.