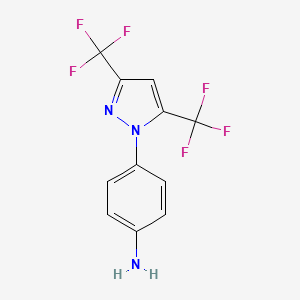
4-(3,5-Bis(trifluoromethyl)-1H-pyrazol-1-yl)phenylamine
Overview
Description
“4-(3,5-Bis(trifluoromethyl)-1H-pyrazol-1-yl)phenylamine” is a chemical compound that has been studied for its potential applications in various fields . It is a derivative of pyrazole, a class of organic compounds characterized by a five-membered aromatic ring with three carbon atoms and two nitrogen atoms .
Synthesis Analysis
The synthesis of “4-(3,5-Bis(trifluoromethyl)-1H-pyrazol-1-yl)phenylamine” involves complex chemical reactions . The process typically involves the reaction of the pyrazole derivative with other reagents under controlled conditions .Molecular Structure Analysis
The molecular structure of “4-(3,5-Bis(trifluoromethyl)-1H-pyrazol-1-yl)phenylamine” is characterized by the presence of a pyrazole ring substituted with a phenylamine group and two trifluoromethyl groups . The exact structure can be determined using techniques such as X-ray crystallography .Chemical Reactions Analysis
“4-(3,5-Bis(trifluoromethyl)-1H-pyrazol-1-yl)phenylamine” can participate in various chemical reactions. For instance, it can react with other compounds to form new derivatives with potential applications in pharmaceuticals and agrochemicals .Physical And Chemical Properties Analysis
The physical and chemical properties of “4-(3,5-Bis(trifluoromethyl)-1H-pyrazol-1-yl)phenylamine” can be determined using various analytical techniques. For instance, its density and refractive index can be measured .Scientific Research Applications
Antimicrobial Agents
CRAC intermediate 2 has demonstrated promising antimicrobial activity. Researchers have synthesized a series of pyrazole derivatives containing this compound, which exhibit potent growth inhibition against drug-resistant bacteria. Notably, some of these derivatives have minimum inhibitory concentration (MIC) values as low as 0.25 µg/mL against planktonic Gram-positive bacteria . These findings suggest its potential as an antibiotic candidate.
Bactericidal Activity Against MRSA
Methicillin-resistant Staphylococcus aureus (MRSA) is a notorious pathogen responsible for nosocomial infections. CRAC intermediate 2 derivatives, such as compounds 11, 28, and 29, have been identified as bactericidal agents against MRSA persisters. These compounds exhibit impressive efficacy, with minimum biofilm eradication concentration (MBEC) values as low as 1 µg/mL against S. aureus biofilms . Their ability to combat MRSA highlights their clinical relevance.
Biofilm Eradication
CRAC intermediate 2 derivatives also show promise in eradicating bacterial biofilms. Biofilms contribute to antibiotic resistance and chronic infections. Compounds 11, 28, and 29 effectively target S. aureus biofilms, making them potential candidates for biofilm-specific therapies .
Pharmaceutical Synthesis
The optically active isomer of CRAC intermediate 2, specifically (S)-3,5-bis(trifluoromethyl)phenyl ethanol, plays a crucial role in pharmaceutical synthesis. For instance, it serves as an intermediate in the production of lipid-lowering agents . Its versatile applications underscore its importance in drug development.
NK1 Antagonists
®-[3,5-bis(trifluoromethyl)phenyl]ethanol (®-3,5-BTPE), derived from CRAC intermediate 2, is utilized in compounding NK1 antagonists. These antagonists have therapeutic potential in various conditions, including chemotherapy-induced nausea and vomiting .
Chemical Biology and Medicinal Chemistry
Researchers continue to explore CRAC intermediate 2 and its derivatives for their interactions with biological targets. Their unique chemical structure and biological activity make them valuable tools for understanding cellular processes and designing novel therapeutic agents.
Safety and Hazards
Future Directions
The future research directions for “4-(3,5-Bis(trifluoromethyl)-1H-pyrazol-1-yl)phenylamine” could involve further exploration of its potential applications in various fields, such as pharmaceuticals and agrochemicals . Additionally, more studies could be conducted to fully understand its mechanism of action and to optimize its synthesis process .
Mechanism of Action
Target of Action
The primary target of 4-(3,5-Bis(trifluoromethyl)-1H-pyrazol-1-yl)phenylamine, also known as CRAC Intermediate 2, is the Calcium Release-Activated Calcium (CRAC) channel . The CRAC channel is a highly Ca2±selective store-operated channel expressed in various tissues, including T cells and mast cells . It plays a crucial role in regulating critical cellular processes such as gene expression, motility, and the secretion of inflammatory mediators .
Mode of Action
It is known that the compound interacts with the crac channels, potentially modulating their activity . This modulation could involve preventing the accumulation of dangerously high levels of calcium caused by a disease insult, while also modulating immune-cell function to counter the inflammatory response associated with the same condition .
Biochemical Pathways
The biochemical pathways affected by CRAC Intermediate 2 are primarily related to calcium signaling. The compound’s interaction with the CRAC channels affects the influx of calcium ions (Ca2+) into the cell . This can influence various downstream effects, including the regulation of gene expression, cell proliferation, secretion of inflammatory mediators, and cell migration .
Result of Action
The result of CRAC Intermediate 2’s action is the modulation of calcium signaling within the cell. By interacting with the CRAC channels, the compound can potentially protect cells from the harmful effects of excessive intracellular calcium levels . This can lead to a reduction in the expression of proinflammatory proteins, such as cytokines by immune cells, which can help to mitigate serious inflammatory disorders .
properties
IUPAC Name |
4-[3,5-bis(trifluoromethyl)pyrazol-1-yl]aniline | |
---|---|---|
Source | PubChem | |
URL | https://pubchem.ncbi.nlm.nih.gov | |
Description | Data deposited in or computed by PubChem | |
InChI |
InChI=1S/C11H7F6N3/c12-10(13,14)8-5-9(11(15,16)17)20(19-8)7-3-1-6(18)2-4-7/h1-5H,18H2 | |
Source | PubChem | |
URL | https://pubchem.ncbi.nlm.nih.gov | |
Description | Data deposited in or computed by PubChem | |
InChI Key |
XOXBUERZFCPKDR-UHFFFAOYSA-N | |
Source | PubChem | |
URL | https://pubchem.ncbi.nlm.nih.gov | |
Description | Data deposited in or computed by PubChem | |
Canonical SMILES |
C1=CC(=CC=C1N)N2C(=CC(=N2)C(F)(F)F)C(F)(F)F | |
Source | PubChem | |
URL | https://pubchem.ncbi.nlm.nih.gov | |
Description | Data deposited in or computed by PubChem | |
Molecular Formula |
C11H7F6N3 | |
Source | PubChem | |
URL | https://pubchem.ncbi.nlm.nih.gov | |
Description | Data deposited in or computed by PubChem | |
DSSTOX Substance ID |
DTXSID10372386 | |
Record name | 4-[3,5-Bis(trifluoromethyl)-1H-pyrazol-1-yl]aniline | |
Source | EPA DSSTox | |
URL | https://comptox.epa.gov/dashboard/DTXSID10372386 | |
Description | DSSTox provides a high quality public chemistry resource for supporting improved predictive toxicology. | |
Molecular Weight |
295.18 g/mol | |
Source | PubChem | |
URL | https://pubchem.ncbi.nlm.nih.gov | |
Description | Data deposited in or computed by PubChem | |
Solubility |
12.9 [ug/mL] (The mean of the results at pH 7.4) | |
Record name | SID26727736 | |
Source | Burnham Center for Chemical Genomics | |
URL | https://pubchem.ncbi.nlm.nih.gov/bioassay/1996#section=Data-Table | |
Description | Aqueous solubility in buffer at pH 7.4 | |
Product Name |
4-(3,5-Bis(trifluoromethyl)-1H-pyrazol-1-yl)phenylamine | |
CAS RN |
123066-64-8 | |
Record name | 4-[3,5-Bis(trifluoromethyl)-1H-pyrazol-1-yl]aniline | |
Source | EPA DSSTox | |
URL | https://comptox.epa.gov/dashboard/DTXSID10372386 | |
Description | DSSTox provides a high quality public chemistry resource for supporting improved predictive toxicology. | |
Retrosynthesis Analysis
AI-Powered Synthesis Planning: Our tool employs the Template_relevance Pistachio, Template_relevance Bkms_metabolic, Template_relevance Pistachio_ringbreaker, Template_relevance Reaxys, Template_relevance Reaxys_biocatalysis model, leveraging a vast database of chemical reactions to predict feasible synthetic routes.
One-Step Synthesis Focus: Specifically designed for one-step synthesis, it provides concise and direct routes for your target compounds, streamlining the synthesis process.
Accurate Predictions: Utilizing the extensive PISTACHIO, BKMS_METABOLIC, PISTACHIO_RINGBREAKER, REAXYS, REAXYS_BIOCATALYSIS database, our tool offers high-accuracy predictions, reflecting the latest in chemical research and data.
Strategy Settings
Precursor scoring | Relevance Heuristic |
---|---|
Min. plausibility | 0.01 |
Model | Template_relevance |
Template Set | Pistachio/Bkms_metabolic/Pistachio_ringbreaker/Reaxys/Reaxys_biocatalysis |
Top-N result to add to graph | 6 |
Feasible Synthetic Routes
Disclaimer and Information on In-Vitro Research Products
Please be aware that all articles and product information presented on BenchChem are intended solely for informational purposes. The products available for purchase on BenchChem are specifically designed for in-vitro studies, which are conducted outside of living organisms. In-vitro studies, derived from the Latin term "in glass," involve experiments performed in controlled laboratory settings using cells or tissues. It is important to note that these products are not categorized as medicines or drugs, and they have not received approval from the FDA for the prevention, treatment, or cure of any medical condition, ailment, or disease. We must emphasize that any form of bodily introduction of these products into humans or animals is strictly prohibited by law. It is essential to adhere to these guidelines to ensure compliance with legal and ethical standards in research and experimentation.