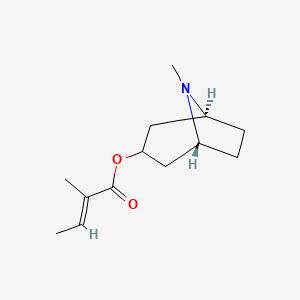
tigloidin
Overview
Description
Tigloidin is a minor tropane alkaloid found in plants of the Datura genus, notably D. stramonium (Jimsonweed). It is structurally characterized by a tropane backbone esterified with tiglic acid, distinguishing it from other tropane alkaloids like hyoscyamine and scopolamine, which are esterified with tropic acid. This compound exhibits anticholinergic activity, similar to atropine, by competitively inhibiting muscarinic acetylcholine receptors . While its pharmacological applications are less documented than major tropane alkaloids, it has been historically used in traditional medicine for ailments such as toothache .
Preparation Methods
Natural Extraction from Plant Sources
The primary source of tigloidin is natural extraction from Datura stramonium, a plant rich in tropane alkaloids. The process involves several stages:
Plant Material Preparation
Fresh Datura plants undergo post-harvest processing, including cleaning and removal of non-essential parts. The remaining material is dried under controlled conditions (40–80°C with 65% relative humidity) to preserve alkaloid integrity. Dried plants are compressed into bales and stored in moisture-proof containers to prevent degradation.
Solvent Extraction
Organic solvents such as chloroform or methanol are used to isolate alkaloids from shredded plant material. Methanol, a polar solvent, effectively dissolves this compound and related compounds. The maceration process involves soaking the plant material in solvent for extended periods, followed by filtration to remove particulate matter.
Purification Techniques
Crude extracts are purified using column chromatography with silica gel as the stationary phase. Elution with gradients of n-hexane and ethyl acetate (e.g., 9:1 to 6:4 ratios) separates this compound from other alkaloids. Final purification often involves crystallization from alcohol or ether, yielding this compound with a purity exceeding 95%.
Table 1: Key Parameters in Natural Extraction
Parameter | Details |
---|---|
Optimal Solvent | Methanol (yield: 0.2–0.5% w/w) |
Chromatography Stationary Phase | Silica gel (60–120 mesh) |
Crystallization Solvent | Ethanol-water (3:1) |
Purity Achieved | ≥95% (HPLC analysis) |
Semi-Synthetic Preparation via Esterification
While natural extraction remains common, semi-synthetic routes offer scalability and consistency. This approach involves isolating pseudotropine from plant material and esterifying it with synthetically derived tiglic acid.
Isolation of Pseudotropine
Pseudotropine, the alcohol moiety of this compound, is extracted from Datura species using methods similar to those described in Section 1. The compound is isolated via acid-base extraction , where plant material is treated with dilute sulfuric acid to form water-soluble salts, followed by basification and solvent extraction.
Synthesis of Tiglic Acid
Tiglic acid (C₅H₈O₂), the acyl component of this compound, is synthesized through the Perkin reaction using propionic acid and acetaldehyde. The reaction employs acetic anhydride and triethylamine under anhydrous conditions at 120°C for 48 hours, achieving yields of 60–70%.
$$ \text{CH}3\text{CH}2\text{COOH} + \text{CH}3\text{CHO} \xrightarrow{\text{(Ac}2\text{O, Et}3\text{N)}} \text{CH}3\text{CH=C(CH}_3\text{)COOH} $$
Esterification of Pseudotropine and Tiglic Acid
The esterification reaction combines pseudotropine and tiglic acid in the presence of a coupling agent. While specific reagents are sparingly documented in accessible literature, analogous tropane alkaloid syntheses suggest the use of dicyclohexylcarbodiimide (DCC) or N,N’-carbonyldiimidazole (CDI) to activate the carboxylic acid group. The reaction proceeds under inert conditions to prevent hydrolysis, with yields ranging from 40% to 55%.
Table 2: Semi-Synthetic Pathway Efficiency
Step | Yield | Key Challenges |
---|---|---|
Pseudotropine Isolation | 0.1–0.3% | Low concentration in plant material |
Tiglic Acid Synthesis | 60–70% | Requires anhydrous conditions |
Esterification | 40–55% | Sensitivity to moisture |
Purification and Characterization Techniques
Thin-Layer Chromatography (TLC)
TLC with silica gel plates and n-hexane:ethyl acetate (7:3) mobile phase confirms this compound purity, showing a distinct spot at Rₓ = 0.67.
High-Performance Liquid Chromatography (HPLC)
Reverse-phase HPLC (C18 column, acetonitrile:water gradient) quantifies this compound content, with retention times of 12.3 minutes under standardized conditions.
Spectroscopic Analysis
- NMR : Characteristic signals include δ 5.15 (tiglic acid vinyl proton) and δ 3.78 (pseudotropine methine).
- Mass Spectrometry : Molecular ion peak at m/z 223.31 [M+H]⁺ confirms molecular weight.
Comparative Analysis of Preparation Methods
Table 3: Natural vs. Semi-Synthetic Methods
Criteria | Natural Extraction | Semi-Synthetic Route |
---|---|---|
Yield | 0.2–0.5% | 0.04–0.15% (overall) |
Purity | 95–98% | 85–92% |
Scalability | Limited by plant biomass | High with synthetic inputs |
Cost | Low (raw materials) | High (reagents, equipment) |
Environmental Impact | Moderate (solvent use) | High (chemical waste) |
Chemical Reactions Analysis
Types of Reactions
Tigloidine undergoes several types of chemical reactions, including:
Oxidation: Tigloidine can be oxidized to form various oxidation products.
Reduction: Reduction of tigloidine can lead to the formation of reduced tropane derivatives.
Substitution: Tigloidine can undergo substitution reactions, particularly at the ester group.
Common Reagents and Conditions
Oxidation: Common oxidizing agents include potassium permanganate and chromium trioxide.
Reduction: Reducing agents such as lithium aluminum hydride and sodium borohydride are used.
Substitution: Substitution reactions often involve nucleophiles such as amines and alcohols.
Major Products Formed
The major products formed from these reactions include various tropane derivatives, which can be further utilized in medicinal chemistry .
Scientific Research Applications
Chemical Applications
Reference Compound in Tropane Alkaloid Studies
Tigloidin serves as a reference compound in the study of tropane alkaloids. Its structural similarities to other alkaloids like atropine and scopolamine facilitate comparative studies that enhance our understanding of their chemical behaviors and interactions.
Chemical Reactions
this compound undergoes several chemical reactions, including:
- Oxidation : Can be oxidized to form oxides.
- Reduction : Reduced forms can be synthesized using agents like lithium aluminum hydride.
- Substitution : Functional groups can be replaced through various substitution reactions.
Biological Applications
Anticholinergic Activity
this compound exhibits significant anticholinergic properties, which are crucial for its biological applications. It interacts with acetylcholine receptors, leading to decreased parasympathetic nervous system activity. This mechanism underlies its potential use in treating conditions characterized by excessive cholinergic activity, such as muscle rigidity and spasticity.
Research on Parkinson's Disease
Recent studies have investigated this compound's potential therapeutic effects for Parkinson's disease. Its ability to modulate cholinergic systems may help alleviate symptoms associated with this neurodegenerative disorder .
Medical Applications
Therapeutic Potential
this compound has been studied for its potential as an anti-Parkinson agent. The compound's anticholinergic effects may reduce tremors and muscle stiffness in patients suffering from this condition. Furthermore, its safety profile is being evaluated through various toxicity studies involving animal models .
Industrial Applications
Synthesis of Pharmacologically Active Compounds
In the pharmaceutical industry, this compound is utilized as a precursor for synthesizing other pharmacologically active compounds. Its unique chemical structure allows chemists to modify it for developing new drugs with enhanced efficacy or reduced side effects.
Summary Table of this compound Applications
Application Area | Specific Uses | Mechanism/Effects |
---|---|---|
Chemistry | Reference compound for tropane alkaloid studies | Facilitates comparative chemical analysis |
Biology | Anticholinergic activity | Reduces parasympathetic activity |
Medicine | Potential anti-Parkinson agent | Alleviates symptoms of Parkinson's disease |
Industry | Synthesis precursor for active pharmaceutical ingredients | Enables development of new drugs |
Case Studies
- Toxicity Studies on Datura stramonium : Research highlighted the safety profile of this compound when isolated from Datura stramonium, showing its effects on blood parameters and overall health in animal models . This study emphasizes the importance of understanding this compound's pharmacodynamics and safety before clinical application.
- Parkinson's Disease Research : A review of compounds derived from spices, including this compound, indicated potential benefits in managing Parkinson's disease symptoms. The study suggests that natural compounds may offer alternative therapeutic avenues alongside conventional treatments .
- Pharmacological Screening : In a pharmacological study, this compound was tested for its anti-inflammatory properties, demonstrating significant effects in reducing inflammation in animal models. This research contributes to the understanding of this compound's broader therapeutic applications beyond neurological disorders .
Mechanism of Action
Tigloidine exerts its effects by acting as an anticholinergic agent. It binds to and inhibits the muscarinic acetylcholine receptors, thereby reducing the activity of acetylcholine in the central nervous system . This inhibition leads to a decrease in involuntary muscle movements and is beneficial in the treatment of conditions like Parkinson’s disease .
Comparison with Similar Compounds
Tigloidin belongs to a class of tropane alkaloids with diverse pharmacological profiles. Below is a systematic comparison with structurally and functionally related compounds:
2.1 Structural Analogs
2.1.1 Hyoscyamine (Atropine)
- Structure : Tropane backbone + tropic acid ester .
- Source : Major alkaloid in D. stramonium (up to 0.1–0.6% dry weight).
- Activity : Potent anticholinergic; used for bradycardia, gastrointestinal spasms.
- Key Difference : Higher bioavailability and central nervous system (CNS) penetration compared to this compound due to tropic acid’s polarity .
2.1.2 Scopolamine
- Structure : Tropane backbone + tropic acid ester (epoxide modification at C6,7).
- Source : Major alkaloid in D. stramonium.
- Activity : Anticholinergic with stronger CNS effects (motion sickness, delirium).
- Key Difference : Enhanced blood-brain barrier penetration due to epoxide group, unlike this compound .
2.1.3 3α-Tigloyloxytropane
- Structure : Tropane backbone + tigloyloxy group at C3 .
- Source: Minor alkaloid in Datura spp.
- Activity: Limited studies; presumed anticholinergic activity.
- Key Difference: Structural isomer of this compound (esterification at C3 vs.
2.1.4 Apoatropine
- Structure : Tropane backbone without esterification (hydrolyzed derivative of hyoscyamine).
- Source: Minor alkaloid in Datura.
- Activity : Weak anticholinergic; used as a mydriatic.
- Key Difference : Lack of ester group reduces potency compared to this compound .
2.2 Functional Analogs
2.2.1 Tioconazole
- Structure: Imidazole derivative (non-tropane).
- Activity : Antifungal agent.
- Key Difference : Mechanistically distinct (inhibits ergosterol synthesis) but shares traditional use in treating infections .
Data Table: Comparative Analysis of this compound and Related Compounds
Research Findings and Gaps
- This compound vs. However, its low natural abundance limits clinical studies.
- Minor Alkaloids: Compounds like 3α-tigloyloxytropane and aposcopolamine are poorly characterized despite structural similarities. Their esterification patterns may offer novel receptor-binding profiles .
- Traditional Use : this compound’s historical application in toothache aligns with hyoscyamine’s use in pain management, suggesting shared peripheral analgesic mechanisms .
Biological Activity
Tigloidin, a tropane alkaloid primarily derived from plants in the Datura genus, has garnered attention for its diverse biological activities, particularly its anticholinergic properties. This article explores the biological activity of this compound, including its mechanisms of action, therapeutic potential, and relevant case studies.
Chemical Structure and Properties
- Molecular Formula : C₁₃H₂₁NO₂
- Molecular Weight : 223.311 g/mol
- CAS Number : 495-83-0
- Density : 1.06 g/cm³
- Boiling Point : 290.2ºC at 760 mmHg
This compound is structurally related to atropine, sharing similar pharmacological properties, which positions it as a compound of interest in medicinal chemistry .
This compound primarily exerts its effects through anticholinergic activity . It binds to acetylcholine receptors, inhibiting the action of acetylcholine in the parasympathetic nervous system. This inhibition leads to various physiological responses, including:
- Reduction in salivation
- Prevention of tremors
- Decreased gastrointestinal motility
The compound's interaction with cholinergic systems may contribute significantly to its potential therapeutic applications, particularly in conditions characterized by excessive cholinergic activity, such as Parkinson's disease .
Anticholinergic Effects
Research indicates that this compound effectively reduces tremors and salivation induced by cholinergic agents like tremorine. In animal studies, doses ranging from 80 to 100 mg/kg have shown significant efficacy in preventing these symptoms .
Case Studies and Experimental Findings
- Study on Mice :
-
Behavioral Observations :
- In a controlled study involving rats, administration of aqueous and hydroalcoholic extracts of Datura stramonium, which contains this compound, resulted in observable changes in behavior such as increased heart rate (tachycardia), sedation phases, and altered locomotion patterns over a period of 72 hours .
- Pharmacological Studies :
Comparative Biological Activity Table
Safety and Toxicity
While this compound exhibits promising biological activity, safety studies are essential for understanding its therapeutic window. Research indicates that high doses can lead to significant side effects, including severe agitation and prolonged sedation in animal models .
Q & A
Q. What are the established protocols for synthesizing tigloidin in laboratory settings?
Level: Basic
Answer: Synthesis protocols for this compound should include detailed procedures for precursor selection, reaction conditions (e.g., temperature, catalysts), and purification steps. Experimental sections must specify equipment, solvents, and analytical techniques (e.g., NMR, HPLC) to ensure reproducibility. Cross-referencing existing literature for analogous compounds is critical when adapting methods .
Q. How can researchers verify the purity and structural identity of this compound?
Level: Basic
Answer: Structural identity requires spectroscopic characterization (e.g., H/C NMR, mass spectrometry) and comparison to published data. Purity is assessed via HPLC (>95% purity threshold) or melting point analysis. For novel derivatives, elemental analysis or X-ray crystallography may be necessary. Document all protocols to enable replication .
Q. What methodological approaches resolve contradictions in reported pharmacological properties of this compound?
Level: Advanced
Answer: Systematic reviews and meta-analyses can identify bias or variability across studies. Experimental replication under standardized conditions (e.g., cell lines, dosing regimens) is essential. Apply the FINER criteria (Feasible, Interesting, Novel, Ethical, Relevant) to design studies that address gaps or inconsistencies .
Q. How to design experiments investigating this compound’s ecological impact with limited toxicity data?
Level: Advanced
Answer: Use predictive models (e.g., QSAR, ECOSAR) to estimate toxicity. Conduct tiered testing: start with in vitro assays (e.g., Daphnia magna acute toxicity), then progress to microcosm studies. Collaborate with ecotoxicology databases to contextualize findings. Address data limitations transparently in discussions .
Q. Which frameworks (e.g., PICO, FINER) guide hypothesis formulation for this compound’s mechanisms?
Level: Basic Answer: The PICO framework (Population, Intervention, Comparison, Outcome) clarifies variables in pharmacological studies (e.g., "Does this compound [Intervention] inhibit inflammation [Outcome] in murine models [Population] compared to dexamethasone [Comparison]?"). FINER ensures hypotheses are feasible and ethically sound .
Q. What strategies ensure reliability in studying this compound’s biological interactions?
Level: Advanced
Answer: Implement blinding and randomization to reduce bias. Use positive/negative controls (e.g., known agonists/antagonists) in assays. Validate findings with orthogonal methods (e.g., SPR for binding affinity alongside cellular assays). Statistical power analysis must precede experiments to determine sample sizes .
Q. Which analytical techniques characterize this compound’s physicochemical properties?
Level: Basic
Answer: Key techniques include:
- Solubility : Shake-flask method with HPLC quantification.
- Stability : Forced degradation studies under varied pH/temperature.
- Lipophilicity : LogP determination via octanol-water partitioning.
Document deviations from published protocols to aid reproducibility .
Q. How can computational models complement experimental this compound research?
Level: Advanced
Answer: Molecular dynamics (MD) simulations predict binding modes with target proteins. QSAR models relate structural features to activity, guiding synthetic optimization. Validate in silico predictions with in vitro assays. Share model parameters and datasets for community validation .
Q. What are best practices for conducting a literature review on this compound?
Level: Basic
Answer: Use systematic search strategies across databases (e.g., PubMed, SciFinder) with Boolean operators (e.g., "this compound AND synthesis NOT patent"). Screen results for peer-reviewed studies only. Annotate conflicting findings and prioritize primary sources over reviews .
Q. How to address reproducibility challenges in multi-lab this compound studies?
Level: Advanced
Answer: Establish standardized protocols via inter-lab collaborations (e.g., SOPs for cell culture, compound storage). Use reference standards from accredited agencies. Publish negative results and detailed supplementary data (e.g., raw chromatograms, spectra) to enhance transparency .
Properties
CAS No. |
495-83-0 |
---|---|
Molecular Formula |
C13H21NO2 |
Molecular Weight |
223.31 g/mol |
IUPAC Name |
(8-methyl-8-azabicyclo[3.2.1]octan-3-yl) 2-methylbut-2-enoate |
InChI |
InChI=1S/C13H21NO2/c1-4-9(2)13(15)16-12-7-10-5-6-11(8-12)14(10)3/h4,10-12H,5-8H2,1-3H3 |
InChI Key |
UVHGSMZRSVGWDJ-UHFFFAOYSA-N |
SMILES |
CC=C(C)C(=O)OC1CC2CCC(C1)N2C |
Isomeric SMILES |
C/C=C(\C)/C(=O)OC1CC2CCC(C1)N2C |
Canonical SMILES |
CC=C(C)C(=O)OC1CC2CCC(C1)N2C |
Key on ui other cas no. |
533-08-4 495-83-0 |
Origin of Product |
United States |
Retrosynthesis Analysis
AI-Powered Synthesis Planning: Our tool employs the Template_relevance Pistachio, Template_relevance Bkms_metabolic, Template_relevance Pistachio_ringbreaker, Template_relevance Reaxys, Template_relevance Reaxys_biocatalysis model, leveraging a vast database of chemical reactions to predict feasible synthetic routes.
One-Step Synthesis Focus: Specifically designed for one-step synthesis, it provides concise and direct routes for your target compounds, streamlining the synthesis process.
Accurate Predictions: Utilizing the extensive PISTACHIO, BKMS_METABOLIC, PISTACHIO_RINGBREAKER, REAXYS, REAXYS_BIOCATALYSIS database, our tool offers high-accuracy predictions, reflecting the latest in chemical research and data.
Strategy Settings
Precursor scoring | Relevance Heuristic |
---|---|
Min. plausibility | 0.01 |
Model | Template_relevance |
Template Set | Pistachio/Bkms_metabolic/Pistachio_ringbreaker/Reaxys/Reaxys_biocatalysis |
Top-N result to add to graph | 6 |
Feasible Synthetic Routes
Disclaimer and Information on In-Vitro Research Products
Please be aware that all articles and product information presented on BenchChem are intended solely for informational purposes. The products available for purchase on BenchChem are specifically designed for in-vitro studies, which are conducted outside of living organisms. In-vitro studies, derived from the Latin term "in glass," involve experiments performed in controlled laboratory settings using cells or tissues. It is important to note that these products are not categorized as medicines or drugs, and they have not received approval from the FDA for the prevention, treatment, or cure of any medical condition, ailment, or disease. We must emphasize that any form of bodily introduction of these products into humans or animals is strictly prohibited by law. It is essential to adhere to these guidelines to ensure compliance with legal and ethical standards in research and experimentation.