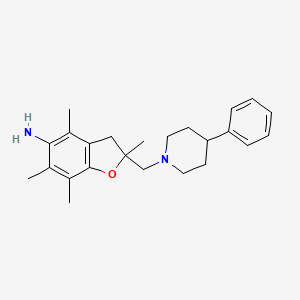
Lipid peroxidation inhibitor 1
Overview
Description
Lipid peroxidation inhibitor 1 (CAS: 142873-41-4) is a synthetic small-molecule compound with the chemical name 2,4,6,7-tetramethyl-2-[(4-phenylpiperidin-1-yl)methyl]-3H-1-benzofuran-5-amine. It exhibits potent inhibitory activity against lipid peroxidation, with an IC50 of 0.07 μM in experimental models . The compound features a benzofuran core substituted with a phenylpiperidine group, which enhances its lipophilicity and membrane permeability. Preclinical studies highlight its efficacy in mitigating oxidative stress in models of neurotoxicity and hyperlocomotion induced by methamphetamine (MAP) .
Preparation Methods
Synthetic Routes and Reaction Conditions
The synthesis of lipid peroxidation inhibitor 1 involves several steps, typically starting with the preparation of the core structure, followed by the introduction of functional groups that enhance its inhibitory activity. The reaction conditions often include the use of specific catalysts, solvents, and temperature controls to ensure the desired product is obtained with high purity and yield.
Industrial Production Methods
In an industrial setting, the production of this compound is scaled up using optimized synthetic routes that are cost-effective and environmentally friendly. This often involves continuous flow reactors and automated systems to maintain consistent quality and efficiency.
Chemical Reactions Analysis
Types of Reactions
Lipid peroxidation inhibitor 1 undergoes various chemical reactions, including:
Oxidation: The compound can be oxidized under specific conditions, leading to the formation of oxidized derivatives.
Reduction: It can also undergo reduction reactions, often resulting in the regeneration of the active inhibitor.
Substitution: The compound can participate in substitution reactions where functional groups are replaced by other groups, potentially altering its inhibitory properties.
Common Reagents and Conditions
Common reagents used in these reactions include oxidizing agents like hydrogen peroxide, reducing agents such as sodium borohydride, and various catalysts that facilitate substitution reactions. The conditions typically involve controlled temperatures, pH levels, and solvent environments to achieve the desired outcomes.
Major Products Formed
The major products formed from these reactions include various oxidized, reduced, and substituted derivatives of this compound, each with potentially different levels of inhibitory activity.
Scientific Research Applications
Cancer Therapy
- Ferroptosis Induction : Recent studies highlight the potential of Lip-1 in enhancing ferroptosis as a therapeutic strategy against tumors. By inhibiting lipid peroxidation, Lip-1 may sensitize cancer cells to therapies that induce ferroptosis, making it a valuable adjunct in cancer treatment .
- Combination Therapies : Lip-1 has shown promise when used in combination with other anticancer agents. For instance, combining Lip-1 with agents that induce oxidative stress can lead to synergistic effects in tumor suppression .
Neuroprotection
- Neurological Disorders : Research indicates that Lip-1 can protect neuronal cells from oxidative stress-induced damage. For example, in models of neurodegeneration, Lip-1 has been effective in reducing lipid peroxidation and improving cell viability .
Regulation of Reactive Oxygen Species (ROS)
- Antioxidant Properties : Lip-1's ability to inhibit lipid peroxidation positions it as a potent antioxidant. This property is crucial in various conditions where ROS play a detrimental role, including inflammation and ischemia-reperfusion injury .
Table: Overview of Key Studies on Lipid Peroxidation Inhibitor 1
Mechanism of Action
Lipid peroxidation inhibitor 1 exerts its effects by neutralizing free radicals and preventing the initiation and propagation of lipid peroxidation. It targets specific molecular pathways involved in oxidative stress, including the inhibition of reactive oxygen species and the stabilization of cell membranes. The compound interacts with various enzymes and proteins that play a role in the oxidative stress response, thereby protecting cells from damage.
Comparison with Similar Compounds
Structural and Functional Overview
Lipid peroxidation inhibitors are categorized based on their mechanisms:
- Radical scavengers (e.g., Trolox, Ferrostatin-1).
- Iron chelators (e.g., Deferoxamine, curcuminoids).
- Enzyme-targeted inhibitors (e.g., GPX4 activators).
Below is a comparative analysis of key compounds:
Table 1: Comparative Analysis of Lipid Peroxidation Inhibitors
Mechanistic Differences
- This compound : Unique benzofuran-piperidine structure enhances membrane penetration. Its high potency (IC50 = 0.07 μM) suggests strong binding to peroxidation intermediates or catalytic sites, though exact targets are unconfirmed .
- Ferrostatin-1 : Specifically inhibits ferroptosis by neutralizing lipid radicals in membranes. Shows tissue-specific efficacy, e.g., rescues corneal transparency by blocking PRDX1-regulated peroxidation .
- Tannic Acid: Combines iron chelation and radical trapping, outperforming DFO in Alzheimer’s models. Synergizes with endogenous antioxidants like GPX4 .
- Curcuminoids: Primarily act via iron chelation, independent of methoxy or phenolic groups. Demonstrated neuroprotection in aging and neurodegenerative models .
Efficacy in Disease Models
Biological Activity
Lipid peroxidation is a critical biochemical process involving the oxidative degradation of lipids, particularly polyunsaturated fatty acids (PUFAs). This process is closely associated with various pathological conditions, including inflammation, neurodegenerative diseases, and cancer. Lipid Peroxidation Inhibitor 1 (LPI-1) has emerged as a significant compound in mitigating the detrimental effects of lipid peroxidation by inhibiting reactive oxygen species (ROS) and their associated pathways. This article delves into the biological activity of LPI-1, supported by data tables, case studies, and detailed research findings.
LPI-1 functions primarily by interrupting the lipid peroxidation chain reaction initiated by ROS. The process can be divided into three stages:
- Initiation : Hydroxyl radicals remove hydrogen from lipids, generating lipid radicals.
- Propagation : Lipid radicals react with oxygen to form lipid peroxy radicals, which further react with other lipids, perpetuating the cycle.
- Termination : Antioxidants such as vitamin E donate hydrogen to lipid peroxy radicals, halting the chain reaction.
LPI-1 acts effectively during the termination phase by enhancing the antioxidant defenses within cells, thereby reducing the formation of harmful lipid hydroperoxides and aldehydes like malondialdehyde (MDA) and 4-hydroxynonenal (4-HNE) .
Role in Disease Prevention
The inhibition of lipid peroxidation is crucial in preventing cellular damage associated with various diseases:
- Cancer : Increased lipid peroxidation has been linked to tumor progression. LPI-1's ability to reduce lipid peroxides can enhance the efficacy of immunotherapies by preserving T cell function in tumor microenvironments .
- Neurodegenerative Diseases : Lipid peroxidation products can induce apoptosis in neuronal cells. LPI-1 may protect against oxidative stress-induced neuronal death, offering potential therapeutic benefits for conditions like Alzheimer's disease .
- Cardiovascular Diseases : By mitigating oxidative stress and inflammation, LPI-1 may play a protective role against atherosclerosis and other cardiovascular conditions .
Study 1: LPI-1 in Cancer Therapy
A study demonstrated that LPI-1 administration in a pancreatic ductal adenocarcinoma mouse model resulted in reduced lipid peroxidation levels and improved tumor regression. The accumulation of lipid peroxides was correlated with enhanced antitumor immunity, indicating that LPI-1 could be an effective adjunct in cancer therapies .
Study 2: Neuroprotective Effects
In vitro studies on neuronal cell cultures exposed to oxidative stress showed that treatment with LPI-1 significantly reduced markers of lipid peroxidation and improved cell viability. These findings suggest that LPI-1 may have neuroprotective properties, potentially beneficial for neurodegenerative disorders .
Data Tables
Parameter | Control Group | LPI-1 Treatment Group |
---|---|---|
Lipid Peroxide Levels (µM) | 25.3 ± 3.5 | 8.7 ± 2.0 |
Cell Viability (%) | 55 ± 5 | 85 ± 4 |
Tumor Volume (mm³) | 150 ± 20 | 80 ± 15 |
Table 1: Comparison of lipid peroxide levels and cell viability between control and LPI-1 treated groups.
Research Findings
Recent studies highlight the multifaceted roles of lipid peroxidation products in cellular signaling:
- 4-HNE as a Signaling Molecule : At low concentrations, 4-HNE promotes adaptive responses and enhances antioxidant capacity; however, at elevated levels, it induces apoptosis .
- Immune Modulation : Lipid peroxidation influences immune cell activation; for instance, T cells exposed to high levels of lipid peroxides exhibit impaired activation due to oxidative stress .
Q & A
Basic Research Questions
Q. What are the critical structural features of Lipid Peroxidation Inhibitor 1 (LPi1) that determine its inhibitory activity?
LPi1 (C₂₄H₃₂N₂O) contains a 2,4,6,7-tetramethylbenzofuran core substituted with a 4-phenylpiperidinylmethyl group. Structure-activity relationship (SAR) studies indicate that the ortho-methyl groups and the distal phenyl group on the piperidine ring are essential for its IC₅₀ of 0.07 μM in lipid peroxidation assays . Comparative analysis with analogs lacking these groups (e.g., compounds 26m, o, p) showed reduced activity, suggesting steric and electronic stabilization of reactive lipid radicals .
Q. How is the efficacy of LPi1 determined in vitro, and what controls are required?
Standard protocols involve:
- Substrate preparation : Linoleic acid (8 mM) and AAPH (20 mM) as peroxidation initiators .
- Inhibitor treatment : LPi1 dissolved in DMSO (final concentration ≤0.1% v/v) at 0.01–1 μM .
- Quantification : Malondialdehyde (MDA) levels via thiobarbituric acid (TBA) assay at 532 nm or fluorescent probes like C11 BODIPY 581/591 (ex/em: 581/591 nm) .
- Controls : Vehicle (DMSO-only), positive controls (e.g., NAC for ROS inhibition), and blank reactions without substrate .
Q. What are the limitations of using MDA as a lipid peroxidation biomarker in LPi1 studies?
MDA-TBA adducts may form non-specifically with other aldehydes (e.g., 4-HNE). Validate results with orthogonal methods:
- LC-MS lipidomics : Untargeted profiling of oxidized phospholipids (e.g., PC(14:1/14:1)) using LipidIMMS Analyzer .
- 4-HNE ELISA : Specific quantification of 4-hydroxynonenal adducts .
Advanced Research Questions
Q. How can contradictory data on LPi1’s efficacy across models (e.g., hepatic vs. neuronal) be resolved?
Discrepancies may arise from:
- Tissue-specific redox environments : Hepatic cells (e.g., HepG2) exhibit higher baseline CYP4A11 activity, which synergizes with lipid peroxidation . Pre-treat with CYP4A11 inhibitors (e.g., HET0016) to isolate LPi1’s effects .
- Membrane composition : Neuronal membranes are enriched in polyunsaturated fatty acids (PUFAs). Use lipidomics to correlate LPi1’s IC₅₀ with PUFA peroxidation rates .
Q. What experimental designs are optimal for studying LPi1’s synergistic effects with ROS scavengers?
- Dose-matrix approach : Combine LPi1 (0.01–1 μM) with NAC (1–10 mM) or Trolox (10–100 μM) in FFA-stimulated HepG2 cells .
- Endpoint multiplexing : Measure MDA (lipid peroxidation), intracellular ROS (DCFH-DA fluorescence), and apoptosis (Annexin V/PI) .
- Statistical analysis : Two-way ANOVA to assess interaction effects (GraphPad Prism) .
Q. How does LPi1 modulate enzyme systems indirectly linked to lipid peroxidation (e.g., Na+/K+-ATPase)?
Lipid peroxidation alters membrane-bound enzyme kinetics. For Na+/K+-ATPase:
- Affinity assays : Pre-treat erythrocyte membranes with LPi1 (0.1 μM) and measure ATPase activity using strophanthidin inhibition curves .
- Membrane fluidity : Confirm via fluorescence polarization with DPH probes .
Q. Methodological Guidance for Data Interpretation
Q. How to validate LPi1’s specificity in complex biological systems?
- Competitive inhibition assays : Co-incubate with α-tocopherol (10 μM) to test for additive effects .
- Knockout models : Use CRISPR-Cas9 to delete key peroxidation mediators (e.g., ALOX15) and compare LPi1’s efficacy .
Q. What are the best practices for standardizing LPi1 stock solutions?
Properties
IUPAC Name |
2,4,6,7-tetramethyl-2-[(4-phenylpiperidin-1-yl)methyl]-3H-1-benzofuran-5-amine | |
---|---|---|
Source | PubChem | |
URL | https://pubchem.ncbi.nlm.nih.gov | |
Description | Data deposited in or computed by PubChem | |
InChI |
InChI=1S/C24H32N2O/c1-16-17(2)23-21(18(3)22(16)25)14-24(4,27-23)15-26-12-10-20(11-13-26)19-8-6-5-7-9-19/h5-9,20H,10-15,25H2,1-4H3 | |
Source | PubChem | |
URL | https://pubchem.ncbi.nlm.nih.gov | |
Description | Data deposited in or computed by PubChem | |
InChI Key |
OFJJFTDZPRQPBY-UHFFFAOYSA-N | |
Source | PubChem | |
URL | https://pubchem.ncbi.nlm.nih.gov | |
Description | Data deposited in or computed by PubChem | |
Canonical SMILES |
CC1=C(C2=C(CC(O2)(C)CN3CCC(CC3)C4=CC=CC=C4)C(=C1N)C)C | |
Source | PubChem | |
URL | https://pubchem.ncbi.nlm.nih.gov | |
Description | Data deposited in or computed by PubChem | |
Molecular Formula |
C24H32N2O | |
Source | PubChem | |
URL | https://pubchem.ncbi.nlm.nih.gov | |
Description | Data deposited in or computed by PubChem | |
Molecular Weight |
364.5 g/mol | |
Source | PubChem | |
URL | https://pubchem.ncbi.nlm.nih.gov | |
Description | Data deposited in or computed by PubChem | |
Retrosynthesis Analysis
AI-Powered Synthesis Planning: Our tool employs the Template_relevance Pistachio, Template_relevance Bkms_metabolic, Template_relevance Pistachio_ringbreaker, Template_relevance Reaxys, Template_relevance Reaxys_biocatalysis model, leveraging a vast database of chemical reactions to predict feasible synthetic routes.
One-Step Synthesis Focus: Specifically designed for one-step synthesis, it provides concise and direct routes for your target compounds, streamlining the synthesis process.
Accurate Predictions: Utilizing the extensive PISTACHIO, BKMS_METABOLIC, PISTACHIO_RINGBREAKER, REAXYS, REAXYS_BIOCATALYSIS database, our tool offers high-accuracy predictions, reflecting the latest in chemical research and data.
Strategy Settings
Precursor scoring | Relevance Heuristic |
---|---|
Min. plausibility | 0.01 |
Model | Template_relevance |
Template Set | Pistachio/Bkms_metabolic/Pistachio_ringbreaker/Reaxys/Reaxys_biocatalysis |
Top-N result to add to graph | 6 |
Feasible Synthetic Routes
Disclaimer and Information on In-Vitro Research Products
Please be aware that all articles and product information presented on BenchChem are intended solely for informational purposes. The products available for purchase on BenchChem are specifically designed for in-vitro studies, which are conducted outside of living organisms. In-vitro studies, derived from the Latin term "in glass," involve experiments performed in controlled laboratory settings using cells or tissues. It is important to note that these products are not categorized as medicines or drugs, and they have not received approval from the FDA for the prevention, treatment, or cure of any medical condition, ailment, or disease. We must emphasize that any form of bodily introduction of these products into humans or animals is strictly prohibited by law. It is essential to adhere to these guidelines to ensure compliance with legal and ethical standards in research and experimentation.