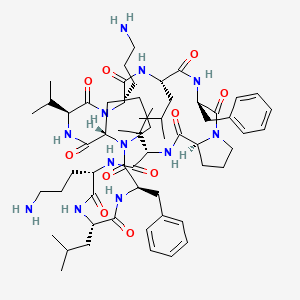
Gramicidin S
Overview
Description
Gramicidin S is a cyclic decapeptide antibiotic produced by the bacterium Brevibacillus brevis. It is composed of two identical pentapeptides joined head-to-tail, forming a ring structure. This compound is effective against a variety of gram-positive and gram-negative bacteria, as well as some fungi . This compound was first discovered in 1941 and has since been studied extensively for its antimicrobial properties .
Mechanism of Action
Target of Action
Gramicidin S, an antibiotic peptide, primarily targets the lipopolysaccharide layer of the cell membrane . It is particularly effective against most gram-positive and few gram-negative bacteria, as well as some fungi .
Mode of Action
The mode of action of this compound involves direct interaction with its targets, leading to rapid antimicrobial activity . The peptide can either form a cationic channel or induce pore formation, altering the ion gradient and ultimately destroying the microorganism .
Pharmacokinetics
Its bactericidal, antipersister, and antibiofilm activities are attributed to its elevated stability, pronounced cell-penetration ability, and effective utilization of multiple modes of antibacterial action .
Result of Action
The result of this compound action is the inhibition of growth and killing of cells of various bacterial strains . It demonstrates potent activity against resistant, persistent, and biofilm cells of staphylococci and enterococci . In mature biofilms, this compound completely kills the cells of all studied strains .
Action Environment
The action of this compound is influenced by environmental factors. Its antimicrobial activity is independent of bacterial resistance patterns, phenotype variations, and biofilm-forming potency .
Biochemical Analysis
Biochemical Properties
Gramicidin S interacts with various biomolecules, primarily through its disruption of the lipid membrane and enhancement of the permeability of the bacterial cytoplasmic membrane . It is synthesized by this compound synthetase , and its biosynthetic pathway consists of two enzymes of nonribosomal peptide synthases (NRPSs), this compound synthetase I (GrsA) and this compound synthetase II (GrsB) .
Cellular Effects
This compound exhibits strong antibiotic activity towards Gram-negative and Gram-positive bacteria and even several pathogenic fungi . The primary mode of action is generally assumed to be the perturbation of lipid packing, resulting in destruction of the membrane integrity and enhancement of the permeability of the lipid bilayer of the bacterial cytoplasmic membrane .
Molecular Mechanism
The molecular mechanism of this compound is not completely understood, but it is generally accepted that it disrupts the lipid membrane and enhances the permeability of the bacterial cytoplasmic membrane . It forms a ring structure composed of five different amino acids, each one used twice within the structure .
Temporal Effects in Laboratory Settings
It is known that this compound has high potential of almost 100% killing mechanisms against most of the gram-positive and few gram-negative bacteria and fungi .
Dosage Effects in Animal Models
Information on the effects of this compound dosage in animal models is limited. It is known that this compound has high potential of almost 100% killing mechanisms against most of the gram-positive and few gram-negative bacteria and fungi .
Metabolic Pathways
This compound is a product obtained from the this compound biosynthetic pathway of non-ribosomal peptide synthetase such as g-S synthetase I and g-S synthetase II .
Transport and Distribution
It is known that this compound is a lipophilic compound that can easily pass through the cell membrane .
Subcellular Localization
This compound is accumulated in nano-globules, which mature by fusion into larger granules and end up within vacuolar structures . These granules serve as energy storage devices, as they contain this compound molecules that are non-covalently attached to alkyl phosphates and protect them from dephosphorylation and premature release of energy .
Preparation Methods
Gramicidin S is synthesized through a nonribosomal peptide synthesis pathway involving two enzymes: this compound synthetase I and this compound synthetase II. These enzymes incorporate and activate specific amino acids, which are then condensed to form the cyclic decapeptide . Industrial production of this compound typically involves fermentation processes using Brevibacillus brevis cultures under controlled conditions .
Chemical Reactions Analysis
Gramicidin S undergoes various chemical reactions, including:
Oxidation: this compound can be oxidized, leading to the formation of different derivatives.
Reduction: Reduction reactions can modify the peptide bonds within this compound.
Substitution: The amino groups in this compound can undergo substitution reactions, such as acetylation, formylation, and carbamylation. Common reagents used in these reactions include oxidizing agents, reducing agents, and acylating agents.
Scientific Research Applications
Gramicidin S has a wide range of scientific research applications:
Comparison with Similar Compounds
Gramicidin S is often compared with other antimicrobial peptides, such as:
Polymyxins: These cyclic peptides also target the bacterial membrane but have different structures and mechanisms of action.
This compound is unique due to its cyclic structure and the presence of uncommon amino acids like ornithine and the atypical stereoisomer of phenylalanine .
Biological Activity
Gramicidin S (GS) is a cyclic decapeptide antibiotic produced by Bacillus brevis. Its unique structure and mechanism of action have made it a subject of extensive research, particularly in the context of its biological activity against various pathogens. This article provides an overview of the biological activity of this compound, focusing on its antimicrobial properties, mechanisms of action, and potential therapeutic applications.
This compound consists of a cyclic beta-sheet structure, which is crucial for its activity. The peptide's sequence is cyclo-FPVOLFPVOL, where certain residues are D-enantiomers. This structure allows this compound to interact with microbial membranes, leading to disruption of membrane integrity and subsequent cell death. The primary mechanisms include:
- Membrane Disruption : GS interacts with the lipid bilayer, leading to depolarization and loss of membrane potential.
- Inhibition of Cellular Processes : It can delocalize peripheral membrane proteins involved in cell division and envelope synthesis, thereby inhibiting bacterial growth even in non-growing cells .
Antimicrobial Activity
This compound exhibits broad-spectrum antimicrobial activity, particularly against Gram-positive bacteria, while showing less efficacy against Gram-negative strains. Its minimal inhibitory concentrations (MICs) and bactericidal effects have been documented extensively:
Microorganism | MIC (μg/mL) | Bactericidal Concentration (5 × MIC) |
---|---|---|
Staphylococcus aureus | 0.5 - 1 | Complete killing at 5 |
Enterococcus faecalis | 0.25 - 0.5 | Rapid bactericidal effect at 5 |
Pseudomonas aeruginosa | 16 - 32 | Limited efficacy |
The effectiveness of GS against biofilms is particularly noteworthy; it has been shown to kill cells within pre-grown biofilms at concentrations ranging from 100-200 μg/mL, preventing regrowth .
Case Studies and Research Findings
- Resistance Mechanisms : Research indicates that this compound remains effective against resistant strains of bacteria due to its multifaceted mechanisms of action. A study showed that GS retained activity against various strains regardless of their resistance patterns .
- Antiviral Properties : Recent studies have explored the antiviral potential of this compound, particularly against SARS-CoV-2. In vitro assays demonstrated significant viral load reduction with GS treatment, showing over 99% viral clearance at 24 hours post-infection .
- Therapeutic Applications : this compound has shown promise in dental applications for treating infections caused by tetracycline-resistant E. faecalis. It demonstrated rapid bactericidal effects on planktonic cells and biofilm-forming strains .
Safety and Toxicity
Despite its potent antimicrobial properties, this compound is known to exhibit hemolytic activity against human red blood cells at certain concentrations. Studies have indicated that modifications to the peptide structure can enhance its specificity for microbial membranes while reducing toxicity to human cells .
Properties
IUPAC Name |
(3R,6S,9S,12S,15S,21R,24S,27S,30S,33S)-9,27-bis(3-aminopropyl)-3,21-dibenzyl-6,24-bis(2-methylpropyl)-12,30-di(propan-2-yl)-1,4,7,10,13,19,22,25,28,31-decazatricyclo[31.3.0.015,19]hexatriacontane-2,5,8,11,14,20,23,26,29,32-decone | |
---|---|---|
Source | PubChem | |
URL | https://pubchem.ncbi.nlm.nih.gov | |
Description | Data deposited in or computed by PubChem | |
InChI |
InChI=1S/C60H92N12O10/c1-35(2)31-43-53(75)67-45(33-39-19-11-9-12-20-39)59(81)71-29-17-25-47(71)55(77)70-50(38(7)8)58(80)64-42(24-16-28-62)52(74)66-44(32-36(3)4)54(76)68-46(34-40-21-13-10-14-22-40)60(82)72-30-18-26-48(72)56(78)69-49(37(5)6)57(79)63-41(23-15-27-61)51(73)65-43/h9-14,19-22,35-38,41-50H,15-18,23-34,61-62H2,1-8H3,(H,63,79)(H,64,80)(H,65,73)(H,66,74)(H,67,75)(H,68,76)(H,69,78)(H,70,77)/t41-,42-,43-,44-,45+,46+,47-,48-,49-,50-/m0/s1 | |
Source | PubChem | |
URL | https://pubchem.ncbi.nlm.nih.gov | |
Description | Data deposited in or computed by PubChem | |
InChI Key |
IUAYMJGZBVDSGL-XNNAEKOYSA-N | |
Source | PubChem | |
URL | https://pubchem.ncbi.nlm.nih.gov | |
Description | Data deposited in or computed by PubChem | |
Canonical SMILES |
CC(C)CC1C(=O)NC(C(=O)N2CCCC2C(=O)NC(C(=O)NC(C(=O)NC(C(=O)NC(C(=O)N3CCCC3C(=O)NC(C(=O)NC(C(=O)N1)CCCN)C(C)C)CC4=CC=CC=C4)CC(C)C)CCCN)C(C)C)CC5=CC=CC=C5 | |
Source | PubChem | |
URL | https://pubchem.ncbi.nlm.nih.gov | |
Description | Data deposited in or computed by PubChem | |
Isomeric SMILES |
CC(C)C[C@H]1C(=O)N[C@@H](C(=O)N2CCC[C@H]2C(=O)N[C@H](C(=O)N[C@H](C(=O)N[C@H](C(=O)N[C@@H](C(=O)N3CCC[C@H]3C(=O)N[C@H](C(=O)N[C@H](C(=O)N1)CCCN)C(C)C)CC4=CC=CC=C4)CC(C)C)CCCN)C(C)C)CC5=CC=CC=C5 | |
Source | PubChem | |
URL | https://pubchem.ncbi.nlm.nih.gov | |
Description | Data deposited in or computed by PubChem | |
Molecular Formula |
C60H92N12O10 | |
Source | PubChem | |
URL | https://pubchem.ncbi.nlm.nih.gov | |
Description | Data deposited in or computed by PubChem | |
DSSTOX Substance ID |
DTXSID6046008 | |
Record name | Gramicidin S | |
Source | EPA DSSTox | |
URL | https://comptox.epa.gov/dashboard/DTXSID6046008 | |
Description | DSSTox provides a high quality public chemistry resource for supporting improved predictive toxicology. | |
Molecular Weight |
1141.4 g/mol | |
Source | PubChem | |
URL | https://pubchem.ncbi.nlm.nih.gov | |
Description | Data deposited in or computed by PubChem | |
CAS No. |
113-73-5 | |
Record name | Gramicidin S [INN] | |
Source | ChemIDplus | |
URL | https://pubchem.ncbi.nlm.nih.gov/substance/?source=chemidplus&sourceid=0000113735 | |
Description | ChemIDplus is a free, web search system that provides access to the structure and nomenclature authority files used for the identification of chemical substances cited in National Library of Medicine (NLM) databases, including the TOXNET system. | |
Record name | Gramicidin S | |
Source | EPA DSSTox | |
URL | https://comptox.epa.gov/dashboard/DTXSID6046008 | |
Description | DSSTox provides a high quality public chemistry resource for supporting improved predictive toxicology. | |
Record name | GRAMICIDIN S | |
Source | FDA Global Substance Registration System (GSRS) | |
URL | https://gsrs.ncats.nih.gov/ginas/app/beta/substances/WHM29QA23F | |
Description | The FDA Global Substance Registration System (GSRS) enables the efficient and accurate exchange of information on what substances are in regulated products. Instead of relying on names, which vary across regulatory domains, countries, and regions, the GSRS knowledge base makes it possible for substances to be defined by standardized, scientific descriptions. | |
Explanation | Unless otherwise noted, the contents of the FDA website (www.fda.gov), both text and graphics, are not copyrighted. They are in the public domain and may be republished, reprinted and otherwise used freely by anyone without the need to obtain permission from FDA. Credit to the U.S. Food and Drug Administration as the source is appreciated but not required. | |
Retrosynthesis Analysis
AI-Powered Synthesis Planning: Our tool employs the Template_relevance Pistachio, Template_relevance Bkms_metabolic, Template_relevance Pistachio_ringbreaker, Template_relevance Reaxys, Template_relevance Reaxys_biocatalysis model, leveraging a vast database of chemical reactions to predict feasible synthetic routes.
One-Step Synthesis Focus: Specifically designed for one-step synthesis, it provides concise and direct routes for your target compounds, streamlining the synthesis process.
Accurate Predictions: Utilizing the extensive PISTACHIO, BKMS_METABOLIC, PISTACHIO_RINGBREAKER, REAXYS, REAXYS_BIOCATALYSIS database, our tool offers high-accuracy predictions, reflecting the latest in chemical research and data.
Strategy Settings
Precursor scoring | Relevance Heuristic |
---|---|
Min. plausibility | 0.01 |
Model | Template_relevance |
Template Set | Pistachio/Bkms_metabolic/Pistachio_ringbreaker/Reaxys/Reaxys_biocatalysis |
Top-N result to add to graph | 6 |
Feasible Synthetic Routes
Q1: How does Gramicidin S exert its antibacterial effect?
A: this compound, a cyclic decapeptide antibiotic, primarily targets bacterial membranes. It disrupts the membrane integrity, leading to increased permeability and leakage of essential intracellular components. [, , ] This ultimately results in bacterial cell death. []
Q2: Does this compound affect Gram-negative and Gram-positive bacteria equally?
A: While this compound exhibits activity against both Gram-positive and Gram-negative bacteria, studies show differential effects. Analogs with altered ring sizes displayed variations in activity against these bacterial types, suggesting potential for targeted development. [] Notably, a 12-residue analog maintained activity against Gram-negative microorganisms and yeast while showing reduced hemolytic activity and decreased potency against Gram-positive bacteria compared to this compound. []
Q3: How does this compound interact with bacterial membranes?
A: this compound interacts with bacterial membranes through a combination of ionic and hydrophobic interactions. [, ] It is suggested that the positively charged ornithine residues of this compound interact with the negatively charged phospholipids in the bacterial membrane. [, ] This interaction is thought to cause phase separation of phospholipids, leading to the formation of “frozen” sites and disruption of the membrane barrier. []
Q4: What is the molecular formula and weight of this compound?
A: The molecular formula of this compound is C60H92N12O10. Its molecular weight is 1141.48 g/mol. []
Q5: What is the secondary structure of this compound?
A: this compound adopts a highly stable, amphipathic, β-sheet conformation. [, , , , ] This structure is stabilized by intramolecular hydrogen bonds and two type II' β-turns, with D-phenylalanine and proline residues occupying positions i+1 and i+2, respectively. [] The β-sheet conformation is crucial for its biological activity. []
Q6: What spectroscopic techniques are commonly used to study this compound?
A6: Several spectroscopic techniques are employed to investigate this compound, including:
- Circular Dichroism (CD) spectroscopy: This technique is widely used to study the secondary structure of this compound and its analogs in various solvents. [, , , , ]
- Nuclear Magnetic Resonance (NMR) spectroscopy: Both 1H NMR and 15N NMR have been used to study the conformation, dynamics, and interactions of this compound with model membranes. [, ]
- Fluorescence Spectroscopy: This technique is utilized to study the interaction of this compound with model membranes and biological systems, often employing fluorescent probes. []
Q7: How do structural modifications affect the activity of this compound?
A7: Modifications to the this compound structure significantly impact its activity. Studies have investigated:
- Ring Size: Analogs with varying ring sizes (4 to 14 residues) revealed a relationship between ring size, secondary structure, and biological activity. [, ] While smaller rings showed reduced activity, larger rings displayed differential activity profiles against Gram-positive and Gram-negative bacteria, as well as varying hemolytic activity. [, ]
- Amino Acid Substitutions: Replacing specific amino acids within the this compound sequence has provided insights into structure-activity relationships. For example:
- Replacing D-phenylalanine with dehydrophenylalanine reinforced the β-sheet conformation and enhanced activity against Gram-positive bacteria. []
- Substituting Pro residues with D-Phe-Pro-D-Val or L-Phe-Pro-D-Val sequences resulted in conformational changes and loss of activity against Gram-positive microorganisms. []
- Replacing valine residues with glycine or alanine resulted in analogs with significantly reduced or abolished activity. [, , ] This highlights the importance of the hydrophobic valine side chains for this compound activity.
- Side Chain Modifications: Altering the charge or hydrophobicity of the ornithine side chains significantly impacted both antibacterial activity and membrane interactions. [, ] Neutralizing the positive charge or introducing negative charges led to a reduction or loss of antibacterial activity, while maintaining a basic character resulted in activity profiles similar to this compound. []
Q8: Are there synergistic effects observed when this compound is combined with other antimicrobial agents?
A: Yes, synergistic effects have been observed when this compound is combined with its acyclic decapeptide analogs, suggesting different modes of action between the cyclic and linear forms. [] Additionally, the presence of heavy metal cations like Cu2+, Ag+, Co2+, and Cd2+ has been shown to enhance the antimicrobial activity of this compound. [] This synergy is thought to be due to increased cationic conductivity of the bacterial cytoplasmic membrane in the presence of these metals. []
Q9: What is known about the stability of this compound?
A: this compound demonstrates good stability under various conditions. It is stable in solution at +4°C for at least three days and at -20°C for up to four weeks when stored in a buffer containing glutathione and glycerol. [] Additionally, this compound maintains its activity over a range of pH values and temperatures.
Q10: What are the potential applications of this compound?
A10: this compound, due to its broad-spectrum antimicrobial activity, holds potential for various applications, including:
- Topical Antibacterial Agent: this compound is currently used in topical applications like wound dressings and ointments for treating superficial bacterial infections. []
- Drug-Resistant Infections: Its unique mechanism of action makes it a promising candidate for combating infections caused by drug-resistant bacteria. []
- Anti-amyloid Activity: Recent research suggests that this compound and its analogs may have potential in treating neurodegenerative diseases by inhibiting the formation of amyloid-β fibrils. []
Q11: What are the current research directions for this compound?
A11: Current research on this compound focuses on:
- Developing Analogs with Improved Properties: This includes enhancing its activity, reducing hemolytic activity, and improving its pharmacokinetic properties. [, , ]
- Understanding its Mechanism of Action: While its interaction with bacterial membranes is established, a deeper understanding of the specific molecular events leading to cell death is crucial for developing more effective analogs. [, , ]
- Exploring New Applications: The potential of this compound in treating drug-resistant infections, biofilms, and even neurodegenerative diseases is an area of active research. [, ]
Q12: What are the limitations of this compound?
A12: Despite its potential, this compound has limitations, including:
- Hemolytic Activity: Its interaction with eukaryotic cell membranes limits its systemic use due to potential toxicity. [, , ]
- Limited Bioavailability: Oral administration of this compound is hampered by poor absorption and rapid degradation in the gastrointestinal tract. []
Q13: What are some alternatives to this compound?
A13: While this compound offers a unique mechanism of action, several alternative antimicrobial peptides and other classes of antibiotics exist, including:
- Other Cyclic Peptides: Tyrocidines, also produced by Bacillus brevis, are cyclic decapeptides with a different amino acid sequence and broader spectrum of activity. []
Q14: What tools and resources are available for research on this compound?
A14: Several resources facilitate research on this compound and related antimicrobial peptides, including:
Q15: What are some of the historical milestones in this compound research?
A15: Key milestones in this compound research include:
- 1944: Discovery and isolation of this compound from Bacillus brevis by Russian scientists. []
- 1950s: Elucidation of the cyclic structure and amino acid sequence of this compound. []
- 1960s-1970s: Extensive research on the mechanism of action, focusing on its interaction with bacterial membranes and effects on membrane permeability. [, , ]
- 1980s-present: Development of synthetic analogs with improved activity and reduced toxicity, exploration of new applications, and investigation of its potential in treating drug-resistant infections and biofilms. [, , , , ]
Q16: What is known about the toxicity of this compound?
A: this compound's primary toxicity concern stems from its hemolytic activity, limiting its systemic use. [, , ] While generally considered safe for topical applications, research continues to explore analogs with reduced hemolytic activity and improve its safety profile for broader applications. []
Disclaimer and Information on In-Vitro Research Products
Please be aware that all articles and product information presented on BenchChem are intended solely for informational purposes. The products available for purchase on BenchChem are specifically designed for in-vitro studies, which are conducted outside of living organisms. In-vitro studies, derived from the Latin term "in glass," involve experiments performed in controlled laboratory settings using cells or tissues. It is important to note that these products are not categorized as medicines or drugs, and they have not received approval from the FDA for the prevention, treatment, or cure of any medical condition, ailment, or disease. We must emphasize that any form of bodily introduction of these products into humans or animals is strictly prohibited by law. It is essential to adhere to these guidelines to ensure compliance with legal and ethical standards in research and experimentation.