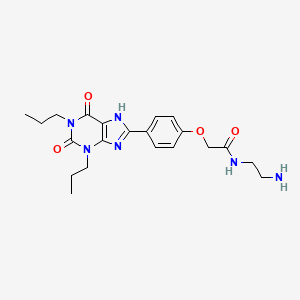
Xanthine amine congener
Overview
Description
Xanthine amine congener is a potent, non-selective adenosine receptor antagonist. It is known for its ability to block the action of adenosine on its receptors, which are involved in various physiological processes such as sleep regulation, cardiovascular function, and neurotransmission . The compound has been widely used in scientific research to study the role of adenosine receptors in different biological systems.
Mechanism of Action
Xanthine amine congener exerts its effects by binding to adenosine receptors and blocking the action of adenosine. This inhibition prevents the activation of downstream signaling pathways that are normally triggered by adenosine binding. The compound has high affinity for multiple adenosine receptor subtypes, including A1, A2A, A2B, and A3 receptors . By blocking these receptors, this compound can modulate various physiological processes, such as neurotransmission, vasodilation, and immune responses .
Similar Compounds:
Theophylline: Another xanthine derivative that acts as a bronchodilator and adenosine receptor antagonist.
Theobromine: A less potent adenosine receptor antagonist found in cocoa and chocolate.
Uniqueness: this compound is unique due to its high potency and non-selective antagonism of multiple adenosine receptor subtypes. This broad-spectrum activity makes it a valuable tool in research for studying the complex roles of adenosine receptors in various physiological and pathological processes .
Advantages and Limitations for Lab Experiments
One advantage of using Xanthine amine congener in lab experiments is its relatively low cost compared to other compounds. This compound is also readily available and easy to synthesize. However, one limitation of using this compound in lab experiments is its potential toxicity at high doses, which can limit its use in certain experiments.
Future Directions
There are many potential future directions for research on Xanthine amine congener. One area of research could focus on the development of this compound analogs with improved therapeutic properties. Another area of research could focus on the potential use of this compound in the treatment of neurodegenerative diseases. Additionally, further research could be conducted on the potential use of this compound in sports medicine and athletic performance enhancement.
Scientific Research Applications
Xanthine amine congener has a wide range of applications in scientific research:
Chemistry: It is used as a tool to study the structure-activity relationships of adenosine receptors and to develop new adenosine receptor antagonists
Biology: The compound is employed to investigate the physiological and pathological roles of adenosine receptors in different biological systems
Medicine: this compound is used in preclinical studies to explore its potential therapeutic effects in conditions such as cardiovascular diseases, neurological disorders, and cancer
Industry: The compound is utilized in the development of new drugs and diagnostic tools targeting adenosine receptors
Safety and Hazards
XAC should be handled with care to avoid dust formation and inhalation . In case of skin or eye contact, it is recommended to wash off with soap and plenty of water or flush eyes with water, respectively . If swallowed, rinse mouth with water . It is also advised to avoid letting the product enter drains .
Biochemical Analysis
Biochemical Properties
In biochemical reactions, Xanthine amine congener interacts with adenosine receptors, specifically A1 and A2B . It reduces adenylate cyclase activity stimulated by 5’-N-ethylcarboxamidoadenosine (NECA) in human platelets . These interactions are crucial in modulating various biochemical processes.
Cellular Effects
This compound influences cell function by interacting with adenosine receptors. It affects cell signaling pathways, gene expression, and cellular metabolism . The compound’s interaction with these receptors can modulate various cellular processes, impacting the overall function and health of the cell .
Molecular Mechanism
At the molecular level, this compound exerts its effects through binding interactions with biomolecules, enzyme inhibition or activation, and changes in gene expression . It acts as an antagonist for adenosine receptors, thereby influencing the activity of these receptors and the downstream cellular processes they regulate .
Temporal Effects in Laboratory Settings
In laboratory settings, the effects of this compound can change over time. Information on the compound’s stability, degradation, and long-term effects on cellular function observed in in vitro or in vivo studies is not currently available .
Dosage Effects in Animal Models
The effects of this compound vary with different dosages in animal models . Specific details about threshold effects, toxic or adverse effects at high doses are not currently available .
Metabolic Pathways
This compound is involved in various metabolic pathways, interacting with enzymes or cofactors . Specific details about the metabolic pathways it is involved in, its effects on metabolic flux or metabolite levels are not currently available .
Transport and Distribution
Details about how this compound is transported and distributed within cells and tissues, including any transporters or binding proteins it interacts with, and any effects on its localization or accumulation, are not currently available .
Subcellular Localization
Information about the subcellular localization of this compound and any effects on its activity or function, including any targeting signals or post-translational modifications that direct it to specific compartments or organelles, is not currently available .
Preparation Methods
Synthetic Routes and Reaction Conditions: The synthesis of xanthine amine congener involves multiple steps, starting from the xanthine core structureOne common synthetic route involves the alkylation of xanthine derivatives followed by amination and subsequent functionalization .
Industrial Production Methods: Industrial production of this compound follows similar synthetic routes but on a larger scale. The process is optimized for yield and purity, often involving the use of advanced techniques such as high-performance liquid chromatography (HPLC) for purification .
Chemical Reactions Analysis
Types of Reactions: Xanthine amine congener undergoes various chemical reactions, including:
Oxidation: The compound can be oxidized to form different derivatives, which may have distinct biological activities.
Reduction: Reduction reactions can modify the functional groups on the xanthine ring, altering its properties.
Substitution: Substitution reactions, particularly nucleophilic substitutions, are common in the modification of this compound
Common Reagents and Conditions:
Oxidation: Common oxidizing agents include hydrogen peroxide and potassium permanganate.
Reduction: Reducing agents such as sodium borohydride and lithium aluminum hydride are often used.
Substitution: Nucleophilic reagents like amines and thiols are frequently employed in substitution reactions
Major Products: The major products formed from these reactions depend on the specific conditions and reagents used. For example, oxidation can lead to the formation of xanthine derivatives with different oxidation states, while substitution reactions can introduce various functional groups to the xanthine ring .
properties
IUPAC Name |
N-(2-aminoethyl)-2-[4-(2,6-dioxo-1,3-dipropyl-7H-purin-8-yl)phenoxy]acetamide | |
---|---|---|
Source | PubChem | |
URL | https://pubchem.ncbi.nlm.nih.gov | |
Description | Data deposited in or computed by PubChem | |
InChI |
InChI=1S/C21H28N6O4/c1-3-11-26-19-17(20(29)27(12-4-2)21(26)30)24-18(25-19)14-5-7-15(8-6-14)31-13-16(28)23-10-9-22/h5-8H,3-4,9-13,22H2,1-2H3,(H,23,28)(H,24,25) | |
Source | PubChem | |
URL | https://pubchem.ncbi.nlm.nih.gov | |
Description | Data deposited in or computed by PubChem | |
InChI Key |
FIQGIOAELHTLHM-UHFFFAOYSA-N | |
Source | PubChem | |
URL | https://pubchem.ncbi.nlm.nih.gov | |
Description | Data deposited in or computed by PubChem | |
Canonical SMILES |
CCCN1C2=C(C(=O)N(C1=O)CCC)NC(=N2)C3=CC=C(C=C3)OCC(=O)NCCN | |
Source | PubChem | |
URL | https://pubchem.ncbi.nlm.nih.gov | |
Description | Data deposited in or computed by PubChem | |
Molecular Formula |
C21H28N6O4 | |
Source | PubChem | |
URL | https://pubchem.ncbi.nlm.nih.gov | |
Description | Data deposited in or computed by PubChem | |
DSSTOX Substance ID |
DTXSID10242595 | |
Record name | 8-(4-((2-Aminoethyl)aminocarbonylmethyloxy)phenyl)-1,3-dipropylxanthine | |
Source | EPA DSSTox | |
URL | https://comptox.epa.gov/dashboard/DTXSID10242595 | |
Description | DSSTox provides a high quality public chemistry resource for supporting improved predictive toxicology. | |
Molecular Weight |
428.5 g/mol | |
Source | PubChem | |
URL | https://pubchem.ncbi.nlm.nih.gov | |
Description | Data deposited in or computed by PubChem | |
CAS RN |
96865-92-8 | |
Record name | 8-(4-((2-Aminoethyl)aminocarbonylmethyloxy)phenyl)-1,3-dipropylxanthine | |
Source | ChemIDplus | |
URL | https://pubchem.ncbi.nlm.nih.gov/substance/?source=chemidplus&sourceid=0096865928 | |
Description | ChemIDplus is a free, web search system that provides access to the structure and nomenclature authority files used for the identification of chemical substances cited in National Library of Medicine (NLM) databases, including the TOXNET system. | |
Record name | 8-(4-((2-Aminoethyl)aminocarbonylmethyloxy)phenyl)-1,3-dipropylxanthine | |
Source | EPA DSSTox | |
URL | https://comptox.epa.gov/dashboard/DTXSID10242595 | |
Description | DSSTox provides a high quality public chemistry resource for supporting improved predictive toxicology. | |
Retrosynthesis Analysis
AI-Powered Synthesis Planning: Our tool employs the Template_relevance Pistachio, Template_relevance Bkms_metabolic, Template_relevance Pistachio_ringbreaker, Template_relevance Reaxys, Template_relevance Reaxys_biocatalysis model, leveraging a vast database of chemical reactions to predict feasible synthetic routes.
One-Step Synthesis Focus: Specifically designed for one-step synthesis, it provides concise and direct routes for your target compounds, streamlining the synthesis process.
Accurate Predictions: Utilizing the extensive PISTACHIO, BKMS_METABOLIC, PISTACHIO_RINGBREAKER, REAXYS, REAXYS_BIOCATALYSIS database, our tool offers high-accuracy predictions, reflecting the latest in chemical research and data.
Strategy Settings
Precursor scoring | Relevance Heuristic |
---|---|
Min. plausibility | 0.01 |
Model | Template_relevance |
Template Set | Pistachio/Bkms_metabolic/Pistachio_ringbreaker/Reaxys/Reaxys_biocatalysis |
Top-N result to add to graph | 6 |
Feasible Synthetic Routes
Q & A
Q1: How does XAC interact with the adenosine A1 receptor?
A1: XAC binds competitively to the adenosine A1 receptor, preventing the binding of adenosine and other agonists. [, , , ] This competitive binding inhibits the downstream signaling cascade associated with A1 receptor activation.
Q2: What are the downstream effects of XAC binding to the A1 receptor?
A2: By blocking A1 receptor activation, XAC can reverse adenosine-mediated effects such as:* Inhibition of adenylyl cyclase activity and subsequent reduction in cAMP levels. [, , , ]* Reduction in potassium conductance leading to membrane depolarization. []* Modulation of neurotransmitter release in various tissues. [, ]
Q3: What is the molecular formula and weight of XAC?
A3: The molecular formula of XAC is C21H28N6O4, and its molecular weight is 428.48 g/mol.
Q4: Is there any available spectroscopic data for XAC?
A4: While specific spectroscopic data isn't explicitly detailed in the provided research, the synthesis and characterization of tritiated XAC ([3H]XAC) for use as a radioligand in binding studies are described. [] This implies the use of techniques like NMR and mass spectrometry for structural confirmation.
Q5: How stable is XAC under various experimental conditions?
A5: While detailed stability data isn't explicitly provided, XAC's use in various experimental settings like cell culture [, , ], isolated organ perfusion [, , ], and in vivo studies [, , ] suggests sufficient stability under those conditions.
Q6: What is the affinity of XAC for different adenosine receptor subtypes?
A6: XAC demonstrates high affinity for the A1 adenosine receptor (Kd values ranging from 0.17 nM in calf brain to 3.0 nM in guinea pig brain). [] It shows significantly lower affinity for A2A and A3 receptors. [, , , ]
Q7: How does the structure of XAC influence its selectivity for the A1 receptor?
A7: While detailed structure-activity relationship (SAR) studies are not extensively described within the provided research, modifications to the xanthine core structure and the amine side chain can significantly impact XAC's affinity and selectivity for different adenosine receptor subtypes. [, ]
Q8: What are the in vivo effects of XAC?
A9: XAC has been shown to:* Attenuate hypoxic vasodilation in isolated guinea pig hearts, suggesting a role for adenosine in mediating this response. []* Increase the occurrence of ischemia-induced ventricular fibrillation in isolated rat hearts, pointing to a potential protective role of endogenous adenosine. []* Inhibit the potentiating effect of adenosine on forskolin-induced cAMP accumulation in PC12 cells. []
Q9: What is the safety profile of XAC?
A9: Detailed toxicological data for XAC is not explicitly provided in the research excerpts.
Q10: What analytical methods are used to characterize and quantify XAC?
A11: Commonly employed techniques include:* Radioligand binding assays using tritiated XAC ([3H]XAC) to determine receptor affinity and density. [, , ]* High-performance liquid chromatography (HPLC) to measure XAC concentrations in biological samples. []
Q11: What research tools and resources are available for studying XAC and adenosine receptors?
A12: Key resources include:* Cell lines expressing various adenosine receptor subtypes. [, , ]* Radiolabeled ligands for binding studies. [, , ]* Selective agonists and antagonists for characterizing receptor subtypes and signaling pathways. [, , , , ]
Disclaimer and Information on In-Vitro Research Products
Please be aware that all articles and product information presented on BenchChem are intended solely for informational purposes. The products available for purchase on BenchChem are specifically designed for in-vitro studies, which are conducted outside of living organisms. In-vitro studies, derived from the Latin term "in glass," involve experiments performed in controlled laboratory settings using cells or tissues. It is important to note that these products are not categorized as medicines or drugs, and they have not received approval from the FDA for the prevention, treatment, or cure of any medical condition, ailment, or disease. We must emphasize that any form of bodily introduction of these products into humans or animals is strictly prohibited by law. It is essential to adhere to these guidelines to ensure compliance with legal and ethical standards in research and experimentation.