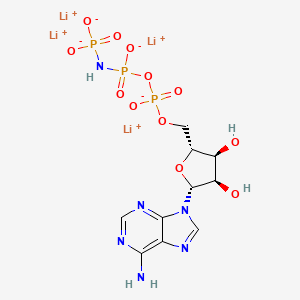
AMP pnp
Overview
Description
Adenosine 5'-(β,γ-imido)triphosphate (AMP-PNP) is a non-hydrolyzable analog of ATP, where the oxygen bridging the β- and γ-phosphate groups is replaced by an imido group (NH). This structural modification renders AMP-PNP resistant to enzymatic hydrolysis, making it invaluable for studying ATP-dependent processes such as molecular motor activity, kinase function, and protein conformational changes . AMP-PNP competes with ATP for binding sites, stabilizing ATP-bound states without enabling energy release through hydrolysis. Its applications span structural biology, enzymology, and cellular biophysics, particularly in elucidating mechanisms of ATPases, helicases, and chaperonins .
Preparation Methods
Synthetic Routes and Reaction Conditions
AMP-PNP (tetralithium) is synthetically prepared through a series of chemical reactions. The synthesis involves the formation of a P-N-P linkage, which replaces the oxygen atom in the β,γ-phosphate bond of ATP with a nitrogen atom. This modification renders the compound non-hydrolyzable. The synthetic route typically includes the following steps:
Formation of Adenosine 5’-Monophosphate (AMP): Adenosine is phosphorylated to form AMP.
Formation of Adenosine 5’-Diphosphate (ADP): AMP is further phosphorylated to form ADP.
Formation of Adenylyl-Imidodiphosphate: ADP is reacted with imidodiphosphate to form adenylyl-imidodiphosphate.
Lithium Salt Formation: The final product is converted to its tetralithium salt form for stability and solubility.
Industrial Production Methods
Industrial production of AMP-PNP (tetralithium) follows similar synthetic routes but on a larger scale. The process involves stringent quality control measures to ensure the purity and stability of the compound. The final product is typically stored at low temperatures to prevent degradation .
Chemical Reactions Analysis
Biochemical Inhibition Mechanisms
AMP-PNP replaces ATP in enzymatic reactions but resists hydrolysis due to its imidodiphosphate group (β,γ-NH substitution). Key inhibitory roles include:
-
ATPase Inhibition : Competitively inhibits mitochondrial F1F0-ATPase (Ki = 0.2–0.5 mM) and sarcoplasmic reticulum Ca²⁺-ATPase, stabilizing pre-hydrolysis states .
-
Kinase and Helicase Blockade : Suppresses ATP-dependent kinases (e.g., DNA topoisomerase II) and helicases (e.g., SV40 large T antigen) by occupying ATP-binding pockets .
-
KATP Channel Blockade : Binds to Kir6.2/SUR1 potassium channels, reducing channel open probability by 80% at 1 mM .
Hydrolysis and Stability
While AMP-PNP is considered non-hydrolyzable, exceptions exist:
-
Limited Hydrolysis : The ncd kinesin motor hydrolyzes AMP-PNP at 1% of ATP’s rate, challenging its classification as fully non-hydrolyzable .
-
Acidic Instability : Rapidly degrades into phosphamide and inorganic phosphate below pH 5 .
Structural Interactions with Enzymes
AMP-PNP induces conformational changes in proteins, as shown by crystallography and spectroscopy:
-
Spa47 ATPase : AMP-PNP binds with low occupancy (<1.0) compared to ATPγS, failing to expel water molecules from the P-loop .
-
Eg5 Kinesin : Stabilizes a pre-hydrolysis state with a two-water cluster in the active site, enabling proton abstraction studies .
-
SERCA1a Ca²⁺-ATPase : Adopts a closed conformation similar to ATP, but alters β-sheet positioning in the P-domain .
Table 1 : Structural Effects of AMP-PNP Binding
Impact on Motor Protein Dynamics
AMP-PNP reveals alternative motility mechanisms in kinesin:
-
Dual Motility Modes : Kinesin exhibits long runs (alternating-site hydrolysis) and short runs (single-site AMP-PNP binding) in ATP/AMP-PNP mixtures .
-
Processivity Maintenance : Sustains processive movement at 50% velocity compared to ATP, despite inhibited hydrolysis .
Key Research Findings:
-
AMP-PNP’s β,γ-imido group prevents γ-phosphate release, making it ideal for trapping ATPase intermediates .
-
Despite its design, slow hydrolysis occurs in select enzymes, necessitating caution in mechanistic studies .
-
Structural studies using AMP-PNP have elucidated water-mediated proton transfer in Eg5 kinesin and BiP chaperone regulation .
Scientific Research Applications
Molecular Biology and Biochemistry
1.1 Role in Protein Dynamics
AMP-PNP has been extensively used to investigate the dynamics of motor proteins such as myosin and dynein. For instance, studies have shown that AMP-PNP can stabilize certain conformations of myosin heads, enabling researchers to analyze their orientation and rotational dynamics under controlled conditions. At low temperatures, AMP-PNP increases orientational disorder and microsecond rotational motion of myosin heads, providing insights into their function in muscle contraction and cellular motility .
1.2 Actin Polymerization Studies
In actin research, AMP-PNP has been utilized to substitute ATP during polymerization studies. This substitution allows scientists to observe changes in actin filament dynamics without the confounding effects of ATP hydrolysis. For example, using electron paramagnetic resonance (EPR) and differential scanning calorimetry (DSC), researchers demonstrated that AMP-PNP influences the internal rigidity of actin systems, leading to longer rotational correlation times and altered thermal transition temperatures . This information is crucial for understanding the mechanics of cell motility.
Cellular Processes
2.1 Flagellar Motility
AMP-PNP has been instrumental in studying flagellar motility in sea urchin sperm. By using demembranated sperm reactivated with AMP-PNP, researchers were able to lock the sperm in "rigor waves," allowing for detailed analysis of the relationship between ATP binding and dynein cross-bridge release. The results indicated that dynein's function is dependent on ATP binding rather than hydrolysis, which has implications for understanding cellular movement mechanisms .
2.2 DNA Repair Mechanisms
The compound has also been applied in studies related to DNA repair processes. In experiments examining RAD51-DNA binding affinity, it was found that AMP-PNP significantly enhanced binding compared to standard conditions with ATP. This suggests that AMP-PNP can be a valuable tool for probing the molecular mechanisms underlying DNA repair and homologous recombination .
Structural Biology
3.1 NMR Studies of Protein Interactions
AMP-PNP has been utilized in solid-state NMR spectroscopy to monitor protein-DNA interactions, particularly with helicases like DnaB from Helicobacter pylori. The binding of AMP-PNP induces conformational changes necessary for ssDNA binding, providing detailed insights into the mechanistic roles of ATP analogs in DNA replication processes . This application is critical for understanding how helicases function at a molecular level.
3.2 Investigating Enzyme Kinetics
Studies using AMP-PNP have highlighted its role in enzyme kinetics by providing a stable substrate for assays involving ATPases. The analog allows researchers to differentiate between ATP binding and hydrolysis effects on enzyme activity, facilitating a clearer understanding of enzyme mechanisms .
Case Studies and Experimental Findings
Mechanism of Action
AMP-PNP (tetralithium) exerts its effects by mimicking ATP while being resistant to hydrolysis. It competitively inhibits ATP-dependent enzyme systems by binding to the active sites of these enzymes. This inhibition prevents the hydrolysis of ATP and stabilizes protein-nucleotide interactions. The compound interacts strongly with proteins like myosin and actomyosin, inhibiting their ATPase activity and affecting cellular processes such as muscle contraction and intracellular transport .
Comparison with Similar Compounds
ATP and ADP
- Structural and Functional Differences: ATP (adenosine triphosphate) serves as the primary energy currency in cells, undergoing hydrolysis to ADP (adenosine diphosphate) to release energy. Unlike ATP, AMP-PNP cannot undergo hydrolysis, allowing researchers to "trap" proteins in pre-hydrolysis states. ADP lacks the γ-phosphate, limiting its utility in studying phosphate-dependent conformational changes .
- Key Studies :
In mitotic spindle studies, AMP-PNP inhibited spindle elongation at 0.2 mM, while ATP hydrolysis drives spindle dynamics. ADP alone fails to induce microtubule stabilization, highlighting AMP-PNP’s unique role in mimicking ATP-bound states .
GTP and Non-Hydrolyzable GTP Analogs (e.g., GTPγS)
- Mechanistic Contrasts :
GTP analogs like GTPγS are used to study GTPases, analogous to AMP-PNP’s role with ATPases. However, GTPγS retains a sulfur substitution in the γ-phosphate, whereas AMP-PNP’s imido group creates a bulkier, more rigid structure. This difference impacts binding pocket interactions and protein activation kinetics . - Research Findings :
In chaperonin studies, AMP-PNP binding induced structural rearrangements similar to ATP but distinct from GTP-bound states, underscoring nucleotide-specific allostery .
ADP-AlFx (Aluminum Fluoride Complexes)
- Functional Overlap and Divergence :
ADP-AlFx mimics the transition state of ATP hydrolysis, whereas AMP-PNP mimics the ATP-bound state. In reverse gyrase studies, AMP-PNP binding showed slower association rates compared to ADP-AlFx, reflecting differences in transition-state stabilization .
Other ATP Analogs (e.g., ATPγS, AMP-PCP)
- Comparative Inhibition Efficacy :
AMP-PNP exhibits higher binding affinity than AMP-PCP (a methylene-modified analog) in kinase assays. ATPγS, which replaces a γ-phosphate oxygen with sulfur, allows partial hydrolysis, unlike AMP-PNP’s complete hydrolysis resistance .
Data Tables
Table 1: Inhibitory Concentrations (IC₅₀) of AMP-PNP in Mitotic Processes
Process | IC₅₀ (mM) | Reference |
---|---|---|
Spindle Elongation | 0.2 | |
Saltatory Motion | 0.8 | |
Chromosome Movement | 8.6 |
Table 2: Structural and Functional Comparison of ATP Analogs
Compound | Hydrolyzable | Key Modification | Primary Use |
---|---|---|---|
ATP | Yes | None | Energy transfer, enzymatic reactions |
AMP-PNP | No | β,γ-imido group | Trapping ATP-bound states |
ADP-AlFx | No | Aluminum fluoride complex | Mimicking hydrolysis transition state |
GTPγS | No | γ-thiophosphate | Studying GTPase activation |
Key Research Findings
- Mitotic Motor Sensitivity : AMP-PNP’s inhibition hierarchy (spindle elongation > saltatory motion > chromosome movement) suggests distinct molecular motors with varying ATPase dependencies .
- Reverse Gyrase Kinetics : AMP-PNP binding to reverse gyrase showed linear concentration dependence, contrasting with ADP-AlFx’s cooperative binding, highlighting mechanistic diversity in ATP-dependent enzymes .
- Chaperonin Conformations : AMP-PNP stabilized the ATP-bound conformation of group II chaperonins, analogous to ATP but distinct from ADP-AlFx, which induced transition-state mimicry .
Q & A
Basic Research Questions
Q. What experimental techniques are commonly used to study AMP-PNP interactions with ATP-dependent enzymes?
AMP-PNP, a non-hydrolyzable ATP analog, is widely used to investigate nucleotide-binding mechanisms. Key methodologies include:
- Isothermal Titration Calorimetry (ITC) : Measures binding affinity and thermodynamics, as demonstrated in the pHipTLp–AMP–PNP complex study .
- X-ray crystallography : Reveals structural details of AMP-PNP bound to enzymes, such as conformational changes in group II chaperonins (e.g., interactions between Lys-161 and catalytic residues in the ATP-binding cavity) .
- Nuclear Magnetic Resonance (NMR) : Useful for dynamic studies of nucleotide-protein interactions, supported by software suites like CNS for multi-method structural analysis .
Q. How does AMP-PNP stabilize ATP-dependent enzymes for structural studies?
AMP-PNP lacks a hydrolyzable γ-phosphate, trapping enzymes in pre-hydrolysis states. This stabilizes transient conformations, enabling high-resolution structural analysis. For example, in the HipBST toxin-antitoxin system, AMP-PNP binding to pHipTLp revealed critical electrostatic interactions and loop rearrangements .
Advanced Research Questions
Q. How can researchers resolve contradictions in structural data when AMP-PNP-bound proteins exhibit conformational variability?
Discrepancies may arise from crystallization conditions or dynamic states. Methodological solutions include:
- Multi-technique validation : Combine crystallography with cryo-EM or NMR to capture flexibility (e.g., NSL loop helical transitions in chaperonins between AMP-PNP and ADP states) .
- Molecular Dynamics (MD) simulations : Model transient states not resolved experimentally .
- Statistical validation : Use software like CNS to refine models against electron density maps and cross-validate with biochemical assays .
Q. What experimental design considerations are critical for ITC studies of AMP-PNP binding kinetics?
Key factors include:
- Buffer optimization : Ensure pH and ionic strength mimic physiological conditions to prevent non-specific interactions .
- Concentration ratios : Use a protein-to-ligand ratio that avoids signal saturation, as seen in pHipTLp–AMP–PNP ITC experiments .
- Data fitting : Employ models accounting for cooperative binding or multiple sites, especially for multi-subunit enzymes.
Q. How can researchers integrate AMP-PNP findings with other nucleotide analogs to elucidate enzymatic mechanisms?
Comparative studies using analogs like ATPγS or ADP-BeF₃ can reveal hydrolysis-dependent conformational shifts. For example:
- Contrast AMP-PNP-bound structures (pre-hydrolysis) with ADP-bound states (post-hydrolysis) to map catalytic trajectories .
- Use kinetic assays (e.g., stopped-flow spectroscopy) to correlate structural changes with enzymatic activity .
Q. Methodological Guidance for Data Interpretation
Q. How should researchers address variability in AMP-PNP binding affinity across homologous enzymes?
- Phylogenetic analysis : Compare binding sites across homologs to identify conserved vs. divergent residues.
- Mutagenesis : Test functional impacts of residues interacting with AMP-PNP’s phosphate groups (e.g., Gly-160 and Lys-161 in chaperonins) .
- Statistical frameworks : Apply ANOVA or Bayesian models to quantify significance in affinity differences .
Q. What strategies mitigate AMP-PNP hydrolysis during prolonged experiments?
- Add stabilizers : Use phosphatase inhibitors (e.g., sodium orthovanadate) in buffers.
- Low-temperature assays : Conduct ITC or crystallography at 4°C to slow enzymatic activity .
- Quality control : Monitor AMP-PNP purity via HPLC (>95% recommended) and validate with mass spectrometry .
Q. Data Presentation and Reproducibility
Q. How should structural data from AMP-PNP studies be presented to ensure reproducibility?
- Deposit coordinates : Submit atomic models to repositories like the PDB (e.g., pHipTLp–AMP–PNP structure) .
- Detail refinement statistics : Include resolution, R-factors, and validation scores (e.g., MolProbity) in supplementary materials .
- Publish raw data : Share ITC thermograms or crystallographic maps via platforms like Zenodo .
Q. Ethical and Reporting Standards
Q. What ethical guidelines apply to studies using AMP-PNP in animal or microbial models?
- Institutional Review : Obtain approval for animal use, specifying species and AMP-PNP dosages .
- Transparency : Disclose conflicts of interest (e.g., commercial AMP-PNP suppliers) and adhere to FAIR data principles .
Properties
IUPAC Name |
tetralithium;[[5-(6-aminopurin-9-yl)-3,4-dihydroxyoxolan-2-yl]methoxy-oxidophosphoryl]oxy-(phosphonatoamino)phosphinate | |
---|---|---|
Details | Computed by Lexichem TK 2.7.0 (PubChem release 2021.05.07) | |
Source | PubChem | |
URL | https://pubchem.ncbi.nlm.nih.gov | |
Description | Data deposited in or computed by PubChem | |
InChI |
InChI=1S/C10H17N6O12P3.4Li/c11-8-5-9(13-2-12-8)16(3-14-5)10-7(18)6(17)4(27-10)1-26-31(24,25)28-30(22,23)15-29(19,20)21;;;;/h2-4,6-7,10,17-18H,1H2,(H,24,25)(H2,11,12,13)(H4,15,19,20,21,22,23);;;;/q;4*+1/p-4 | |
Details | Computed by InChI 1.0.6 (PubChem release 2021.05.07) | |
Source | PubChem | |
URL | https://pubchem.ncbi.nlm.nih.gov | |
Description | Data deposited in or computed by PubChem | |
InChI Key |
FDBDJIGGLGUOPP-UHFFFAOYSA-J | |
Details | Computed by InChI 1.0.6 (PubChem release 2021.05.07) | |
Source | PubChem | |
URL | https://pubchem.ncbi.nlm.nih.gov | |
Description | Data deposited in or computed by PubChem | |
Canonical SMILES |
[Li+].[Li+].[Li+].[Li+].C1=NC(=C2C(=N1)N(C=N2)C3C(C(C(O3)COP(=O)([O-])OP(=O)(NP(=O)([O-])[O-])[O-])O)O)N | |
Details | Computed by OEChem 2.3.0 (PubChem release 2021.05.07) | |
Source | PubChem | |
URL | https://pubchem.ncbi.nlm.nih.gov | |
Description | Data deposited in or computed by PubChem | |
Molecular Formula |
C10H13Li4N6O12P3 | |
Details | Computed by PubChem 2.1 (PubChem release 2021.05.07) | |
Source | PubChem | |
URL | https://pubchem.ncbi.nlm.nih.gov | |
Description | Data deposited in or computed by PubChem | |
DSSTOX Substance ID |
DTXSID20993728 | |
Record name | Tetralithium 9-[5-O-({[(phosphonatoamino)phosphinato]oxy}phosphinato)pentofuranosyl]-9H-purin-6-amine | |
Source | EPA DSSTox | |
URL | https://comptox.epa.gov/dashboard/DTXSID20993728 | |
Description | DSSTox provides a high quality public chemistry resource for supporting improved predictive toxicology. | |
Molecular Weight |
530.0 g/mol | |
Details | Computed by PubChem 2.1 (PubChem release 2021.05.07) | |
Source | PubChem | |
URL | https://pubchem.ncbi.nlm.nih.gov | |
Description | Data deposited in or computed by PubChem | |
CAS No. |
72957-42-7 | |
Record name | Tetralithium 9-[5-O-({[(phosphonatoamino)phosphinato]oxy}phosphinato)pentofuranosyl]-9H-purin-6-amine | |
Source | EPA DSSTox | |
URL | https://comptox.epa.gov/dashboard/DTXSID20993728 | |
Description | DSSTox provides a high quality public chemistry resource for supporting improved predictive toxicology. | |
Retrosynthesis Analysis
AI-Powered Synthesis Planning: Our tool employs the Template_relevance Pistachio, Template_relevance Bkms_metabolic, Template_relevance Pistachio_ringbreaker, Template_relevance Reaxys, Template_relevance Reaxys_biocatalysis model, leveraging a vast database of chemical reactions to predict feasible synthetic routes.
One-Step Synthesis Focus: Specifically designed for one-step synthesis, it provides concise and direct routes for your target compounds, streamlining the synthesis process.
Accurate Predictions: Utilizing the extensive PISTACHIO, BKMS_METABOLIC, PISTACHIO_RINGBREAKER, REAXYS, REAXYS_BIOCATALYSIS database, our tool offers high-accuracy predictions, reflecting the latest in chemical research and data.
Strategy Settings
Precursor scoring | Relevance Heuristic |
---|---|
Min. plausibility | 0.01 |
Model | Template_relevance |
Template Set | Pistachio/Bkms_metabolic/Pistachio_ringbreaker/Reaxys/Reaxys_biocatalysis |
Top-N result to add to graph | 6 |
Feasible Synthetic Routes
Disclaimer and Information on In-Vitro Research Products
Please be aware that all articles and product information presented on BenchChem are intended solely for informational purposes. The products available for purchase on BenchChem are specifically designed for in-vitro studies, which are conducted outside of living organisms. In-vitro studies, derived from the Latin term "in glass," involve experiments performed in controlled laboratory settings using cells or tissues. It is important to note that these products are not categorized as medicines or drugs, and they have not received approval from the FDA for the prevention, treatment, or cure of any medical condition, ailment, or disease. We must emphasize that any form of bodily introduction of these products into humans or animals is strictly prohibited by law. It is essential to adhere to these guidelines to ensure compliance with legal and ethical standards in research and experimentation.