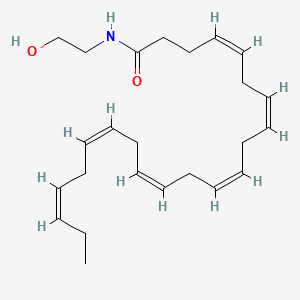
Synaptamide
Overview
Description
Docosahexaenoyl ethanolamide is a bioactive lipid derived from docosahexaenoic acid, an omega-3 fatty acid. It is part of the endocannabinoid system and has been studied for its potential therapeutic effects in various physiological processes, including inflammation, glucose metabolism, and neuroprotection .
Mechanism of Action
Target of Action
Synaptamide, an endogenous metabolite derived from docosahexaenoic acid (DHA), has been identified as a specific ligand to the orphan adhesion G-protein-coupled receptor 110 (GPR110, ADGRF1) . GPR110 is highly expressed in neutrophils and macrophages .
Mode of Action
This compound interacts with its primary target, GPR110, to mediate its effects. It increases intracellular cAMP levels and inhibits the translocation of NF-κB subunit RelA into the nucleus . This interaction results in the suppression of lipopolysaccharide (LPS)-induced proinflammatory cytokine expression . The effects of this compound are abolished when its binding to GPR110 is blocked .
Biochemical Pathways
This compound affects the cAMP/PKA signaling pathway . By binding to GPR110, it enhances cAMP/PKA signaling, leading to the inhibition of NF-κB activation . This results in the suppression of LPS-induced proinflammatory cytokine expression .
Pharmacokinetics
It is known that this compound is an endogenous metabolite of dha . Both unesterified DHA and DHA-lysophosphatidylcholine (DHA-lysoPC), the two major lipid forms that deliver DHA to the brain, are metabolized to this compound in a time- and concentration-dependent manner .
Result of Action
This compound has potent anti-inflammatory effects. It significantly reduces LPS-induced production of TNF-α and NO in cultured microglia cells . It also suppresses LPS-induced neuroinflammation in mice . Furthermore, this compound promotes the anti-inflammatory transformation of the microglial phenotype .
Action Environment
The action of this compound can be influenced by environmental factors such as the presence of inflammation. For instance, in the context of neuroinflammation induced by LPS, this compound exhibits potent anti-inflammatory effects . .
Biochemical Analysis
Biochemical Properties
Synaptamide is synthesized from DHA-containing phosphatidylethanolamine (DHA-PE) through the action of specific phospholipase D (PLD)This compound binds to GPR110, leading to the activation of the cAMP/protein kinase A (PKA) signaling pathway . This interaction promotes neurogenesis, neurite outgrowth, and synaptogenesis, highlighting its role in enhancing brain function and plasticity .
Cellular Effects
This compound exerts various effects on different cell types and cellular processes. In neural cells, it promotes the differentiation of neural stem cells and enhances glutamatergic synaptic activity . This compound also exhibits anti-inflammatory properties by reducing the activation of microglia and astrocytes, which are key players in neuroinflammation . Additionally, this compound influences cell signaling pathways, gene expression, and cellular metabolism by increasing intracellular cAMP levels and activating the cAMP-response element binding protein (CREB) .
Molecular Mechanism
The molecular mechanism of this compound involves its binding to the GAIN domain of GPR110, triggering a conformational change that activates the cAMP/PKA signaling pathway . This activation leads to the phosphorylation of CREB, which in turn regulates the expression of genes involved in neurogenesis and synaptogenesis . This compound also inhibits the nuclear translocation of NF-κB subunit RelA, thereby reducing the expression of pro-inflammatory cytokines .
Temporal Effects in Laboratory Settings
In laboratory settings, this compound has shown stability and sustained effects over time. Studies have demonstrated that this compound can prevent long-term neurodegenerative changes and improve hippocampal plasticity in models of traumatic brain injury . The compound’s anti-inflammatory and neuroprotective effects are maintained over extended periods, indicating its potential for long-term therapeutic use .
Dosage Effects in Animal Models
The effects of this compound vary with different dosages in animal models. At lower doses, this compound effectively reduces neuroinflammation and promotes neurogenesis . Higher doses may lead to adverse effects, such as increased oxidative stress and apoptosis . It is crucial to determine the optimal dosage to maximize therapeutic benefits while minimizing potential toxicity.
Metabolic Pathways
This compound is involved in several metabolic pathways, including the modulation of ketone body and amino acid metabolism . It is synthesized from DHA through the action of enzymes such as N-acyltransferase and NAPE-hydrolyzing phospholipase D . This compound also influences the levels of arachidonic acid-derived eicosanoids and other lipid metabolites, contributing to its anti-inflammatory effects .
Transport and Distribution
Within cells and tissues, this compound is transported and distributed through interactions with specific transporters and binding proteins . It is known to accumulate in the brain, where it exerts its neurogenic and neuroprotective effects . The distribution of this compound is influenced by its binding to GPR110, which facilitates its localization to specific cellular compartments .
Subcellular Localization
This compound is localized to various subcellular compartments, including the plasma membrane and intracellular vesicles . Its activity and function are influenced by post-translational modifications and targeting signals that direct it to specific organelles . The subcellular localization of this compound is crucial for its role in modulating cellular processes and signaling pathways .
Preparation Methods
Synthetic Routes and Reaction Conditions
Docosahexaenoyl ethanolamide can be synthesized through the reaction of docosahexaenoic acid with ethanolamine. This reaction typically involves the use of a coupling agent such as N,N’-dicyclohexylcarbodiimide (DCC) to facilitate the formation of the amide bond . The reaction is usually carried out in an organic solvent like dichloromethane under mild conditions to prevent the degradation of the sensitive polyunsaturated fatty acid.
Industrial Production Methods
Industrial production of docosahexaenoyl ethanolamide often involves enzymatic synthesis using lipases. This method is preferred due to its higher specificity and milder reaction conditions, which help maintain the integrity of the docosahexaenoic acid . The enzymatic process typically involves the use of immobilized lipases in a solvent-free system, making it an environmentally friendly approach.
Chemical Reactions Analysis
Types of Reactions
Docosahexaenoyl ethanolamide undergoes various chemical reactions, including:
Reduction: Reduction reactions are less common but can lead to the formation of saturated ethanolamides.
Common Reagents and Conditions
Oxidation: Common oxidizing agents include hydrogen peroxide and lipoxygenases.
Reduction: Reducing agents like sodium borohydride can be used.
Substitution: Reagents such as acyl chlorides and anhydrides are commonly used for substitution reactions.
Major Products
Hydroxylated derivatives: These are major products of oxidation and have enhanced anti-inflammatory properties.
Saturated ethanolamides: These are products of reduction reactions and have different biological activities compared to the parent compound.
Scientific Research Applications
Docosahexaenoyl ethanolamide has a wide range of scientific research applications:
Comparison with Similar Compounds
Similar Compounds
Anandamide (arachidonoylethanolamide): Another endocannabinoid with similar structure but derived from arachidonic acid.
Eicosapentaenoyl ethanolamide: Derived from eicosapentaenoic acid, another omega-3 fatty acid.
Uniqueness
Docosahexaenoyl ethanolamide is unique due to its origin from docosahexaenoic acid, which provides it with distinct anti-inflammatory and neuroprotective properties compared to other ethanolamides . Its ability to modulate glucose uptake and influence the endocannabinoid system further distinguishes it from similar compounds .
Properties
IUPAC Name |
(4Z,7Z,10Z,13Z,16Z,19Z)-N-(2-hydroxyethyl)docosa-4,7,10,13,16,19-hexaenamide | |
---|---|---|
Source | PubChem | |
URL | https://pubchem.ncbi.nlm.nih.gov | |
Description | Data deposited in or computed by PubChem | |
InChI |
InChI=1S/C24H37NO2/c1-2-3-4-5-6-7-8-9-10-11-12-13-14-15-16-17-18-19-20-21-24(27)25-22-23-26/h3-4,6-7,9-10,12-13,15-16,18-19,26H,2,5,8,11,14,17,20-23H2,1H3,(H,25,27)/b4-3-,7-6-,10-9-,13-12-,16-15-,19-18- | |
Source | PubChem | |
URL | https://pubchem.ncbi.nlm.nih.gov | |
Description | Data deposited in or computed by PubChem | |
InChI Key |
GEEHOLRSGZPBSM-KUBAVDMBSA-N | |
Source | PubChem | |
URL | https://pubchem.ncbi.nlm.nih.gov | |
Description | Data deposited in or computed by PubChem | |
Canonical SMILES |
CCC=CCC=CCC=CCC=CCC=CCC=CCCC(=O)NCCO | |
Source | PubChem | |
URL | https://pubchem.ncbi.nlm.nih.gov | |
Description | Data deposited in or computed by PubChem | |
Isomeric SMILES |
CC/C=C\C/C=C\C/C=C\C/C=C\C/C=C\C/C=C\CCC(=O)NCCO | |
Source | PubChem | |
URL | https://pubchem.ncbi.nlm.nih.gov | |
Description | Data deposited in or computed by PubChem | |
Molecular Formula |
C24H37NO2 | |
Source | PubChem | |
URL | https://pubchem.ncbi.nlm.nih.gov | |
Description | Data deposited in or computed by PubChem | |
DSSTOX Substance ID |
DTXSID50678596 | |
Record name | N-(2-Hydroxyethyl)docosa-4,7,10,13,16,19-hexaenamide | |
Source | EPA DSSTox | |
URL | https://comptox.epa.gov/dashboard/DTXSID50678596 | |
Description | DSSTox provides a high quality public chemistry resource for supporting improved predictive toxicology. | |
Molecular Weight |
371.6 g/mol | |
Source | PubChem | |
URL | https://pubchem.ncbi.nlm.nih.gov | |
Description | Data deposited in or computed by PubChem | |
Physical Description |
Solid | |
Record name | Docosahexaenoyl Ethanolamide | |
Source | Human Metabolome Database (HMDB) | |
URL | http://www.hmdb.ca/metabolites/HMDB0013658 | |
Description | The Human Metabolome Database (HMDB) is a freely available electronic database containing detailed information about small molecule metabolites found in the human body. | |
Explanation | HMDB is offered to the public as a freely available resource. Use and re-distribution of the data, in whole or in part, for commercial purposes requires explicit permission of the authors and explicit acknowledgment of the source material (HMDB) and the original publication (see the HMDB citing page). We ask that users who download significant portions of the database cite the HMDB paper in any resulting publications. | |
CAS No. |
162758-94-3 | |
Record name | Synaptamide | |
Source | CAS Common Chemistry | |
URL | https://commonchemistry.cas.org/detail?cas_rn=162758-94-3 | |
Description | CAS Common Chemistry is an open community resource for accessing chemical information. Nearly 500,000 chemical substances from CAS REGISTRY cover areas of community interest, including common and frequently regulated chemicals, and those relevant to high school and undergraduate chemistry classes. This chemical information, curated by our expert scientists, is provided in alignment with our mission as a division of the American Chemical Society. | |
Explanation | The data from CAS Common Chemistry is provided under a CC-BY-NC 4.0 license, unless otherwise stated. | |
Record name | N-(2-Hydroxyethyl)docosa-4,7,10,13,16,19-hexaenamide | |
Source | EPA DSSTox | |
URL | https://comptox.epa.gov/dashboard/DTXSID50678596 | |
Description | DSSTox provides a high quality public chemistry resource for supporting improved predictive toxicology. | |
Record name | Docosahexaenoyl Ethanolamide | |
Source | Human Metabolome Database (HMDB) | |
URL | http://www.hmdb.ca/metabolites/HMDB0013658 | |
Description | The Human Metabolome Database (HMDB) is a freely available electronic database containing detailed information about small molecule metabolites found in the human body. | |
Explanation | HMDB is offered to the public as a freely available resource. Use and re-distribution of the data, in whole or in part, for commercial purposes requires explicit permission of the authors and explicit acknowledgment of the source material (HMDB) and the original publication (see the HMDB citing page). We ask that users who download significant portions of the database cite the HMDB paper in any resulting publications. | |
Retrosynthesis Analysis
AI-Powered Synthesis Planning: Our tool employs the Template_relevance Pistachio, Template_relevance Bkms_metabolic, Template_relevance Pistachio_ringbreaker, Template_relevance Reaxys, Template_relevance Reaxys_biocatalysis model, leveraging a vast database of chemical reactions to predict feasible synthetic routes.
One-Step Synthesis Focus: Specifically designed for one-step synthesis, it provides concise and direct routes for your target compounds, streamlining the synthesis process.
Accurate Predictions: Utilizing the extensive PISTACHIO, BKMS_METABOLIC, PISTACHIO_RINGBREAKER, REAXYS, REAXYS_BIOCATALYSIS database, our tool offers high-accuracy predictions, reflecting the latest in chemical research and data.
Strategy Settings
Precursor scoring | Relevance Heuristic |
---|---|
Min. plausibility | 0.01 |
Model | Template_relevance |
Template Set | Pistachio/Bkms_metabolic/Pistachio_ringbreaker/Reaxys/Reaxys_biocatalysis |
Top-N result to add to graph | 6 |
Feasible Synthetic Routes
Q1: What is the primary mechanism of action of synaptamide?
A1: this compound exerts its effects primarily by binding to and activating the G-protein coupled receptor 110 (GPR110, also known as ADGRF1). []
Q2: What are the downstream effects of this compound binding to GPR110?
A2: Binding of this compound to GPR110 triggers the cAMP/protein kinase A (PKA) signaling pathway. [] This pathway plays a vital role in neuronal development, survival, and plasticity.
Q3: How does this compound affect axon growth?
A3: this compound has been shown to promote axon growth in cultured cortical neurons. [, ] This effect is mediated through cAMP elevation, and interestingly, is antagonized by Sonic Hedgehog (Shh) signaling via a non-canonical, Smoothened-independent pathway. []
Q4: What is the role of this compound in neurogenesis?
A4: this compound demonstrates potent neurogenic activity by inducing neuronal differentiation of neural stem cells (NSCs). [] This effect is mediated through the activation of the PKA/CREB pathway, a critical regulator of neuronal development. []
Q5: How does this compound contribute to neuroprotection?
A5: this compound displays neuroprotective effects by reducing neuroinflammation, promoting neurogenesis, and improving synaptic plasticity. [, , , , , ] For example, in a mouse model of traumatic brain injury, this compound administration improved cognitive function, reduced neuronal death, and modulated the inflammatory response. []
Q6: What is the molecular formula and weight of this compound?
A6: The molecular formula of this compound is C24H37NO2, and its molecular weight is 371.56 g/mol.
Q7: Is there any spectroscopic data available for this compound?
A7: While specific spectroscopic data is not provided in the provided abstracts, this compound can be characterized using techniques like Nuclear Magnetic Resonance (NMR) spectroscopy and Mass Spectrometry (MS). These methods can provide information about its structure and purity.
Q8: Is there information available on the material compatibility and stability of this compound?
A8: The provided abstracts focus primarily on the biological activity and therapeutic potential of this compound. Further research is needed to fully understand its compatibility with various materials and its stability under different storage conditions.
Q9: Does this compound possess any catalytic properties?
A9: this compound is not known to exhibit catalytic properties. Its biological activity primarily stems from its role as a signaling molecule, specifically as a ligand for GPR110.
Q10: How do structural modifications of this compound affect its activity?
A10: While the provided abstracts do not delve into specific SAR studies, research suggests that the length and saturation of the acyl chain play a crucial role in this compound's binding affinity and biological activity.
Q11: Are there any known this compound analogs with improved potency or selectivity?
A11: One study investigated dimethylthis compound (A8) as a stable analog of this compound. A8 also activates GPR110 and showed efficacy in a model of optic nerve injury. [] This suggests that structural modifications can potentially enhance this compound's therapeutic potential.
Disclaimer and Information on In-Vitro Research Products
Please be aware that all articles and product information presented on BenchChem are intended solely for informational purposes. The products available for purchase on BenchChem are specifically designed for in-vitro studies, which are conducted outside of living organisms. In-vitro studies, derived from the Latin term "in glass," involve experiments performed in controlled laboratory settings using cells or tissues. It is important to note that these products are not categorized as medicines or drugs, and they have not received approval from the FDA for the prevention, treatment, or cure of any medical condition, ailment, or disease. We must emphasize that any form of bodily introduction of these products into humans or animals is strictly prohibited by law. It is essential to adhere to these guidelines to ensure compliance with legal and ethical standards in research and experimentation.