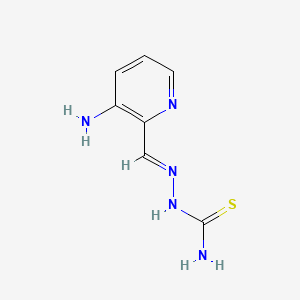
Triapine
Overview
Description
Triapine, also known as 3-aminopyridine-2-carboxaldehyde thiosemicarbazone, is a potent small-molecule inhibitor of ribonucleotide reductase. This enzyme is crucial for DNA synthesis, making this compound a promising candidate for cancer treatment. It has been investigated in multiple clinical trials for its efficacy against various cancers, particularly hematological malignancies .
Mechanism of Action
Target of Action
Triapine, also known as 3-aminopyridine-2-carboxaldehyde thiosemicarbazone, primarily targets the enzyme Ribonucleotide Reductase (RNR) . RNR plays a crucial role in DNA synthesis and repair, and is often overexpressed in multiple cancers . It is responsible for the conversion of ribonucleoside diphosphates to deoxyribonucleotides, which are necessary for DNA synthesis .
Mode of Action
This compound interacts with its target, RNR, by inhibiting its activity . It presents an N-N-S array of donor sites that strongly bind iron, thereby depriving iron-containing enzymes, like RNR, of their prosthetic group . This interaction results in the inhibition of the conversion of ribonucleoside diphosphates to deoxyribonucleotides, thereby disrupting DNA synthesis .
Biochemical Pathways
The primary biochemical pathway affected by this compound is the DNA synthesis pathway. By inhibiting RNR, this compound disrupts the conversion of ribonucleoside diphosphates to deoxyribonucleotides, which are essential for DNA synthesis . This disruption can lead to cell cycle arrest and apoptosis, thereby inhibiting tumor growth .
Pharmacokinetics
Studies suggest that this compound is rapidly metabolized and excreted . The main metabolic reactions observed were dehydrogenation and hydroxylation . The dehydrogenated metabolite M1, identified as a thiadiazole ring-closed oxidation product of this compound, is of particular interest as it loses the crucial chemical property of α-N-heterocyclic thiosemicarbazones to bind metal ions .
Result of Action
The molecular and cellular effects of this compound’s action primarily involve the inhibition of cell proliferation and the induction of apoptosis . For instance, in NF1-associated MPNST cells, RRM2 knockdown and the RRM2 inhibitor this compound were used to assess cell proliferation and apoptosis. The results showed that this compound inhibited cell proliferation and promoted apoptosis and S-phase arrest .
Action Environment
The action, efficacy, and stability of this compound can be influenced by various environmental factors. For instance, the presence of iron in the reaction mixture can affect the potency of this compound . Additionally, the chalcogen atom exchange from S (this compound) to O (O-Triapine) and Se (Se-Triapine) and the methylation of the hydrazonic NH moiety (Me-Triapine) can influence its complexation with Fe(II), Fe(III) and Cu(II) ions and their cytotoxicity .
Biochemical Analysis
Biochemical Properties
Triapine presents an N-N-S array of donor sites that strongly bind iron, robbing iron-containing enzymes of their prosthetic group . It belongs to the family of drugs called ribonucleotide reductase inhibitors . The main metabolic reactions of this compound, observed by electrochemical oxidations, liver microsomes and in vivo samples from mice, were dehydrogenation and hydroxylations .
Cellular Effects
Treatment of tumor cell lines with this compound causes a reduction in one or more of the deoxyribonucleotide triphosphates (dNTPs), resulting in the arrest or slowing of DNA synthesis and cellular proliferation . This compound’s potent inactivation of ribonucleotide reductase arrests cells at the G1/S cell cycle restriction checkpoint and enhances cisplatin–radiation cytotoxicity .
Molecular Mechanism
The mechanism by which this compound inhibits ribonucleotide reductase is similar to that of hydroxyurea. Both agents inhibit the M2 subunit of ribonucleotide reductase by quenching a tyrosyl-free radical . It is believed that the mode of action of this compound is based on the effective inhibition of ribonucleotide reductase as a result of Fe (II) chelation from the diferric-tyrosyl cofactor, followed by the generation of reactive oxygen species (ROS) .
Temporal Effects in Laboratory Settings
The in vivo data of the urine samples revealed very high levels of the metabolites and this compound itself already 15 min after treatment . This clearly indicates that this compound is rapidly metabolised and excreted .
Dosage Effects in Animal Models
In the toxicology studies of this compound, short 15-min i.v. infusions caused emesis in dogs, which was reduced by extending the infusion to 2 h .
Metabolic Pathways
The main metabolic reactions of this compound were dehydrogenation and hydroxylations . The dehydrogenated metabolite M1 was identified as a thiadiazole ring-closed oxidation product of this compound . CYP1A2 metabolism is the major metabolic pathway for this compound .
Transport and Distribution
This compound showed linear pharmacokinetic behavior although interpatient variability was relatively high . Peak concentrations at the 96-mg/m^2/day dose averaged 8 μm, and the mean elimination T1/2 ranged from 35 min to 3 h, with a median value of ∼1 h .
Subcellular Localization
Fluorescence microscopy of living SW480 (colon carcinoma) cells treated with this compound showed that this compound has a striking affinity to the nuclear membrane and to an organelle structure in the cytoplasm, whereas the localization in the nucleus was strongly different .
Preparation Methods
Triapine is synthesized through the condensation of 3-aminopyridine-2-carboxaldehyde with thiosemicarbazide. The reaction typically occurs in an aqueous or alcoholic medium under reflux conditions. The product is then purified through recrystallization .
Industrial production methods for this compound involve similar synthetic routes but on a larger scale. The reaction conditions are optimized for higher yields and purity, often involving continuous flow reactors and advanced purification techniques .
Chemical Reactions Analysis
Triapine undergoes several types of chemical reactions, including:
Oxidation: this compound can be oxidized electrochemically or enzymatically to form various metabolites.
Substitution: This compound can undergo substitution reactions, particularly with metal ions, forming complexes with copper and iron.
Common reagents and conditions for these reactions include electrochemical cells, liver microsomes, and various oxidizing agents. The major products formed from these reactions are typically oxidized or metal-bound derivatives of this compound .
Scientific Research Applications
Triapine has a wide range of scientific research applications:
Comparison with Similar Compounds
Triapine belongs to the class of α-N-heterocyclic thiosemicarbazones, which are known for their ribonucleotide reductase inhibitory activity. Similar compounds include:
- 2-formylpyridine thiosemicarbazone
- 5-hydroxy-2-formylpyridine thiosemicarbazone
- 3-aminopyridine-2-carboxaldehyde thiosemicarbazone derivatives
Compared to these compounds, this compound is unique due to its higher potency and selectivity for ribonucleotide reductase. It has shown promising results in clinical trials, particularly against hematological malignancies, although its efficacy against solid tumors remains limited .
Properties
IUPAC Name |
[(E)-(3-aminopyridin-2-yl)methylideneamino]thiourea | |
---|---|---|
Source | PubChem | |
URL | https://pubchem.ncbi.nlm.nih.gov | |
Description | Data deposited in or computed by PubChem | |
InChI |
InChI=1S/C7H9N5S/c8-5-2-1-3-10-6(5)4-11-12-7(9)13/h1-4H,8H2,(H3,9,12,13)/b11-4+ | |
Source | PubChem | |
URL | https://pubchem.ncbi.nlm.nih.gov | |
Description | Data deposited in or computed by PubChem | |
InChI Key |
XMYKNCNAZKMVQN-NYYWCZLTSA-N | |
Source | PubChem | |
URL | https://pubchem.ncbi.nlm.nih.gov | |
Description | Data deposited in or computed by PubChem | |
Canonical SMILES |
C1=CC(=C(N=C1)C=NNC(=S)N)N | |
Source | PubChem | |
URL | https://pubchem.ncbi.nlm.nih.gov | |
Description | Data deposited in or computed by PubChem | |
Isomeric SMILES |
C1=CC(=C(N=C1)/C=N/NC(=S)N)N | |
Source | PubChem | |
URL | https://pubchem.ncbi.nlm.nih.gov | |
Description | Data deposited in or computed by PubChem | |
Molecular Formula |
C7H9N5S | |
Source | PubChem | |
URL | https://pubchem.ncbi.nlm.nih.gov | |
Description | Data deposited in or computed by PubChem | |
DSSTOX Substance ID |
DTXSID90893923 | |
Record name | 3-Aminopyridine-2-carboxaldehyde thiosemicarbazone | |
Source | EPA DSSTox | |
URL | https://comptox.epa.gov/dashboard/DTXSID90893923 | |
Description | DSSTox provides a high quality public chemistry resource for supporting improved predictive toxicology. | |
Molecular Weight |
195.25 g/mol | |
Source | PubChem | |
URL | https://pubchem.ncbi.nlm.nih.gov | |
Description | Data deposited in or computed by PubChem | |
CAS No. |
143621-35-6 | |
Record name | Triapine | |
Source | ChemIDplus | |
URL | https://pubchem.ncbi.nlm.nih.gov/substance/?source=chemidplus&sourceid=0143621356 | |
Description | ChemIDplus is a free, web search system that provides access to the structure and nomenclature authority files used for the identification of chemical substances cited in National Library of Medicine (NLM) databases, including the TOXNET system. | |
Record name | Triapine | |
Source | DrugBank | |
URL | https://www.drugbank.ca/drugs/DB11940 | |
Description | The DrugBank database is a unique bioinformatics and cheminformatics resource that combines detailed drug (i.e. chemical, pharmacological and pharmaceutical) data with comprehensive drug target (i.e. sequence, structure, and pathway) information. | |
Explanation | Creative Common's Attribution-NonCommercial 4.0 International License (http://creativecommons.org/licenses/by-nc/4.0/legalcode) | |
Record name | 3-Aminopyridine-2-carboxaldehyde thiosemicarbazone | |
Source | EPA DSSTox | |
URL | https://comptox.epa.gov/dashboard/DTXSID90893923 | |
Description | DSSTox provides a high quality public chemistry resource for supporting improved predictive toxicology. | |
Record name | 2-[(3-Aminopyridin-2-yl)methylene]hydrazinecarbothioamide | |
Source | European Chemicals Agency (ECHA) | |
URL | https://echa.europa.eu/information-on-chemicals | |
Description | The European Chemicals Agency (ECHA) is an agency of the European Union which is the driving force among regulatory authorities in implementing the EU's groundbreaking chemicals legislation for the benefit of human health and the environment as well as for innovation and competitiveness. | |
Explanation | Use of the information, documents and data from the ECHA website is subject to the terms and conditions of this Legal Notice, and subject to other binding limitations provided for under applicable law, the information, documents and data made available on the ECHA website may be reproduced, distributed and/or used, totally or in part, for non-commercial purposes provided that ECHA is acknowledged as the source: "Source: European Chemicals Agency, http://echa.europa.eu/". Such acknowledgement must be included in each copy of the material. ECHA permits and encourages organisations and individuals to create links to the ECHA website under the following cumulative conditions: Links can only be made to webpages that provide a link to the Legal Notice page. | |
Record name | OCX-0191 | |
Source | FDA Global Substance Registration System (GSRS) | |
URL | https://gsrs.ncats.nih.gov/ginas/app/beta/substances/U4XIL4091C | |
Description | The FDA Global Substance Registration System (GSRS) enables the efficient and accurate exchange of information on what substances are in regulated products. Instead of relying on names, which vary across regulatory domains, countries, and regions, the GSRS knowledge base makes it possible for substances to be defined by standardized, scientific descriptions. | |
Explanation | Unless otherwise noted, the contents of the FDA website (www.fda.gov), both text and graphics, are not copyrighted. They are in the public domain and may be republished, reprinted and otherwise used freely by anyone without the need to obtain permission from FDA. Credit to the U.S. Food and Drug Administration as the source is appreciated but not required. | |
Synthesis routes and methods
Procedure details
Retrosynthesis Analysis
AI-Powered Synthesis Planning: Our tool employs the Template_relevance Pistachio, Template_relevance Bkms_metabolic, Template_relevance Pistachio_ringbreaker, Template_relevance Reaxys, Template_relevance Reaxys_biocatalysis model, leveraging a vast database of chemical reactions to predict feasible synthetic routes.
One-Step Synthesis Focus: Specifically designed for one-step synthesis, it provides concise and direct routes for your target compounds, streamlining the synthesis process.
Accurate Predictions: Utilizing the extensive PISTACHIO, BKMS_METABOLIC, PISTACHIO_RINGBREAKER, REAXYS, REAXYS_BIOCATALYSIS database, our tool offers high-accuracy predictions, reflecting the latest in chemical research and data.
Strategy Settings
Precursor scoring | Relevance Heuristic |
---|---|
Min. plausibility | 0.01 |
Model | Template_relevance |
Template Set | Pistachio/Bkms_metabolic/Pistachio_ringbreaker/Reaxys/Reaxys_biocatalysis |
Top-N result to add to graph | 6 |
Feasible Synthetic Routes
Disclaimer and Information on In-Vitro Research Products
Please be aware that all articles and product information presented on BenchChem are intended solely for informational purposes. The products available for purchase on BenchChem are specifically designed for in-vitro studies, which are conducted outside of living organisms. In-vitro studies, derived from the Latin term "in glass," involve experiments performed in controlled laboratory settings using cells or tissues. It is important to note that these products are not categorized as medicines or drugs, and they have not received approval from the FDA for the prevention, treatment, or cure of any medical condition, ailment, or disease. We must emphasize that any form of bodily introduction of these products into humans or animals is strictly prohibited by law. It is essential to adhere to these guidelines to ensure compliance with legal and ethical standards in research and experimentation.