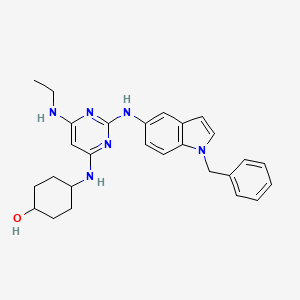
Cdk4/6 Inhibitor IV
Overview
Description
Cyclin-dependent kinase 4/6 (CDK4/6) inhibitors are a class of targeted therapies that block the transition from the G1 to S phase of the cell cycle by inhibiting CDK4/6-cyclin D complexes, thereby suppressing tumor proliferation in hormone receptor-positive (HR+), human epidermal growth factor receptor 2-negative (HER2−) breast cancer . Three FDA-approved inhibitors—palbociclib, ribociclib, and abemaciclib—have revolutionized treatment for advanced breast cancer, though resistance and adverse events (AEs) remain challenges . This article compares these agents and discusses emerging insights relevant to newer compounds like Cdk4/6 Inhibitor IV (hypothetical or under investigation), emphasizing efficacy, safety, resistance mechanisms, and combination strategies.
Preparation Methods
Overview of CDK4/6 Inhibitor IV Synthesis
This compound belongs to the pyrido[2,3-d]pyrimidin-7-one structural family, characterized by a cyclopentyl-substituted core and piperazine-containing side chains. Its synthesis demands precise control over regioselectivity and stereochemistry, particularly during pyrimidine functionalization and spirocyclic intermediate formation. Two primary routes dominate current industrial production: (1) a pyrimidine-based cascade reaction utilizing cyclopentylamine derivatives, and (2) a spirocyclic indole construction via hydrazone intermediates. Both approaches address historical challenges in CDK4/6 inhibitor synthesis, such as the need for cryogenic conditions and column chromatography-dependent purification.
Synthetic Route from Cyclopentylamine-Pyrimidine Intermediate
Nucleophilic Amination of 2,4-Dichloro-5-Acetylpyrimidine
The synthesis commences with the regioselective amination of 2,4-dichloro-5-acetylpyrimidine using cyclopentylamine under mild basic conditions. Triethylamine or potassium carbonate in ethyl acetate facilitates chloride displacement at the C4 position while preserving the C2-chloro substituent. Temperature control (-5°C to 5°C) prevents di-amination byproducts, yielding 2-chloro-4-cyclopentylamino-5-acetylpyrimidine with >85% purity after acid-wash and crystallization.
Lithium Hexamethyldisilazide-Mediated Cyclization
The critical ring-forming step employs lithium hexamethyldisilazide (LiHMDS) in anhydrous tetrahydrofuran at -70°C to 0°C. This strong base deprotonates the acetyl group, enabling intramolecular cyclization with the adjacent pyrimidine nitrogen. The reaction progresses through a ketene intermediate, ultimately generating the pyrido[2,3-d]pyrimidin-7-one core. Post-reaction workup involves saturated ammonium chloride quenching and methyl tert-butyl ether extraction, followed by recrystallization in ethyl acetate to achieve 92-95% purity.
Piperazine Coupling and Salt Formation
The final stages involve Buchwald-Hartwig amination between the C2-chloro intermediate and tert-butyl 4-(6-aminopyridin-3-yl)piperazine-1-carboxylate. Palladium catalysts in dimethylformamide/toluene mixtures at 80-100°C facilitate this carbon-nitrogen bond formation. Subsequent acid hydrolysis (HCl in dioxane) removes the tert-butoxycarbonyl protecting group, yielding the target compound as a hydrochloride salt with an overall yield of 68-72% across four steps.
Spirocyclic Indole-Based Synthesis Approach
Hydrazone Formation with (4-Bromo-2-Fluorophenyl)Hydrazine
Alternative routes prioritize spirocyclic indole moieties, beginning with the condensation of (4-bromo-2-fluorophenyl)hydrazine hydrochloride with cyclopentanone derivatives. This exothermic reaction proceeds in ethanol/water mixtures at 25-40°C, forming a stable hydrazone intermediate detectable by thin-layer chromatography. The crystalline product (mp 142-145°C) requires no column purification, demonstrating 97% purity by HPLC.
Acid-Catalyzed Spirocyclization
Treatment with concentrated sulfuric acid induces Fischer indole synthesis, cyclizing the hydrazone into a spiro[cyclopentane-1,3'-indole] system. The reaction's exothermic nature necessitates temperature control (0-5°C) to prevent over-sulfonation. Neutralization with ammonium hydroxide precipitates the spirocyclic compound, which is isolated via filtration in 89% yield.
Palladium-Catalyzed Cross-Coupling
A Suzuki-Miyaura coupling installs the pyrimidine ring using 2-chloro-5-fluoropyrimidin-4-ylboronic acid. Tetrakis(triphenylphosphine)palladium(0) catalyzes this transformation in degassed toluene/ethanol under microwave irradiation (120°C, 30 min). Post-reaction purification via antisolvent crystallization (n-heptane) affords the bis-aryl product in 94% purity, avoiding silica gel chromatography.
Comparative Analysis of Synthesis Methodologies
The pyrimidine route excels in atom economy but requires stringent temperature control during cyclization. Conversely, the spirocyclic approach benefits from straightforward intermediate isolation but generates acidic waste streams. Both methods successfully eliminate column chromatography, enhancing their industrial viability compared to earlier routes dependent on precious metal catalysts.
Chemical Reactions Analysis
Types of Reactions
CINK4 undergoes several types of chemical reactions, including:
Oxidation: CINK4 can be oxidized under specific conditions to form corresponding oxidized products.
Reduction: Reduction reactions can be employed to modify the functional groups within the molecule.
Substitution: Nucleophilic and electrophilic substitution reactions are common for modifying the indole and pyrimidine moieties.
Common Reagents and Conditions
Oxidation: Common oxidizing agents include potassium permanganate and chromium trioxide.
Reduction: Reducing agents such as sodium borohydride and lithium aluminum hydride are used.
Substitution: Reagents like alkyl halides and acyl chlorides are employed for substitution reactions.
Major Products Formed
The major products formed from these reactions include various derivatives of CINK4 with modified functional groups, which can be further explored for their biological activities .
Scientific Research Applications
The search results do not contain information about a specific compound called "Cdk4/6 Inhibitor IV." However, they do provide extensive information on CDK4/6 inhibitors in general, their applications, and related research. Here's a summary based on the available data:
CDK4/6 Inhibitors: Overview
Cyclin-dependent kinases 4 and 6 (CDK4/6) are key regulators of the cell cycle . CDK4/6 inhibitors have become a standard-of-care treatment, particularly when combined with endocrine therapy, for patients with hormone receptor-positive (HR+), HER2-negative breast cancer . These inhibitors are also being explored in other breast cancer subtypes and clinical scenarios .
FDA Approved CDK4/6 Inhibitors
- Palbociclib
- Ribociclib
- Abemaciclib
These inhibitors have shown significant improvements in progression-free survival in clinical trials .
HR+/HER2- Metastatic Breast Cancer
CDK4/6 inhibitors combined with endocrine therapy are a primary treatment for advanced-stage HR+/HER2- breast cancer . CDER researchers at the FDA have found that adding a CDK4/6 inhibitor to hormonal agents consistently improves progression-free survival across patient subgroups .
HR+/HER2+ Metastatic Breast Cancer
The addition of a CDK4/6 inhibitor to standard anti-HER2 and endocrine therapy has shown to significantly extend the time before the disease progresses . The PATINA trial demonstrated that adding palbociclib to anti-HER2 therapy and endocrine therapy resulted in a 15-month improvement in progression-free survival for patients with HR+/HER2+ metastatic breast cancer .
Other Breast Cancer Subtypes
CDK4/6 inhibitors are under investigation for other breast cancer subtypes, often in combination with targeted therapies or immunotherapies . Preclinical studies suggest that CDK4/6 inhibitors can boost tumor cell immunogenicity, leading to the exploration of combinations with immune checkpoint inhibitors .
Cell Cycle Inhibition
CDK4/6 inhibitors work by inhibiting the cell cycle, which can induce cellular senescence .
Immunomodulatory Effects
Research indicates that CDK4/6 inhibition can enhance antitumor immunity . They promote IL-2 secretion, enhance T-cell activation and tumor infiltration, and cooperate with anti-PD-1 antibodies . While CDK4/6 inhibitors may decrease T-cell proliferation, they increase tumor infiltration and activation of effector T-cells .
Overcoming Resistance
The clinical success of CDK4/6 inhibitors has led to increased research into mechanisms of acquired resistance . Genomic alterations in several genes have been associated with resistance to CDK4/6 inhibition in patients with HR+HER2- breast cancer . Current research efforts are exploring potential treatment combinations to overcome these resistance mechanisms .
Novel Selective Inhibitors
Mechanism of Action
CINK4 exerts its effects by specifically inhibiting the kinase activity of CDK4. This inhibition prevents the phosphorylation of the retinoblastoma protein (pRb), a crucial step in cell cycle progression. By blocking this pathway, CINK4 induces cell cycle arrest and apoptosis in cancer cells. The molecular targets include CDK4/cyclin D1 complexes, and the pathways involved are primarily related to cell cycle regulation .
Comparison with Similar Compounds
Efficacy Comparison of CDK4/6 Inhibitors
Clinical Trial Outcomes
Common AEs and Real-World Data
- Cdk4/6 Inhibitor IV : Preclinical models (e.g., Ly2835219, a research analog of abemaciclib) show cell cycle-dependent/independent antitumor activity with gemcitabine synergy . Future agents may prioritize reduced QT prolongation and hepatotoxicity .
Resistance Mechanisms and Overcoming Strategies
Key Resistance Pathways
Upstream Signaling : Activation of PI3K/AKT/mTOR or ER pathways bypasses CDK4/6 inhibition .
Downstream Bypass : Loss of Rb function or cyclin E overexpression .
Epigenetic Alterations : HDAC-mediated p21 suppression or lncRNA TROJAN-driven CDK2 activation .
Strategies to Overcome Resistance
- Combination Therapies: PI3K/AKT inhibitors: Synergistic with CDK4/6 inhibitors in Rb-positive models . Immunotherapy: Enhanced tumor immunogenicity observed in preclinical studies .
- Biomarker-Driven Approaches : Rb status, cyclin D1 amplification, and CDK4 phosphorylation predict sensitivity .
Biological Activity
Cdk4/6 inhibitors, particularly Cdk4/6 Inhibitor IV (commonly referred to as PD 0332991), have emerged as significant therapeutic agents in oncology, especially for hormone receptor-positive breast cancer. This article explores the biological activity of this compound, including its mechanisms of action, clinical efficacy, resistance mechanisms, and comparative studies with other inhibitors.
Cdk4/6 inhibitors primarily function by blocking the phosphorylation of the retinoblastoma (RB) protein. This inhibition prevents the progression of the cell cycle from the G1 phase to the S phase, effectively inducing cell cycle arrest in cancer cells. The inhibition of Cdk4 and Cdk6 leads to a decrease in E2F target gene expression, which is crucial for DNA synthesis and cell proliferation .
Key Points:
- Target : Cdk4 and Cdk6
- Primary Action : Prevents RB phosphorylation
- Outcome : Induces G1/S phase cell cycle arrest
Clinical Efficacy
This compound has demonstrated substantial clinical activity in various malignancies, particularly in metastatic breast cancer. The combination of Cdk4/6 inhibitors with endocrine therapy has shown improved progression-free survival (PFS) rates compared to endocrine therapy alone. For instance, pivotal trials have indicated that treatment regimens involving PD 0332991 significantly enhance overall response rates and PFS in both premenopausal and postmenopausal women with HR+/HER2-negative metastatic breast cancer .
Table 1: Summary of Clinical Trials Involving PD 0332991
Study Name | Population | Treatment Regimen | Median PFS (months) | Overall Response Rate (%) |
---|---|---|---|---|
PALOMA-1 | HR+/HER2- MBC | PD 0332991 + letrozole | 20.2 | 60 |
PALOMA-3 | HR+/HER2- MBC | PD 0332991 + anastrozole | 24.8 | 63 |
MONALEESA-2 | HR+/HER2- MBC | Ribociclib + letrozole | 23.8 | 58 |
Resistance Mechanisms
Despite its efficacy, resistance to Cdk4/6 inhibitors can develop. Intrinsic resistance is often linked to alterations in the RB pathway or mutations in genes such as RB1, AURKA, and CCNE2. Acquired resistance may arise through various mechanisms, including upregulation of alternative signaling pathways that bypass the need for CDK activity .
Common Resistance Mechanisms:
- Genetic Alterations : Mutations in RB1 and other critical genes
- Pathway Activation : Enhanced signaling through RAS/ERK pathways
- Cellular Adaptation : Changes in cellular microenvironment or tumor heterogeneity
Comparative Studies
Recent studies have compared the biological activity of PD 0332991 with other Cdk4/6 inhibitors like ribociclib and abemaciclib. These studies highlight differences in selectivity, potency, and side effect profiles among these agents.
Table 2: Comparative Biological Activity of Cdk4/6 Inhibitors
Inhibitor | IC50 (nM) against CDK4 | IC50 (nM) against CDK6 | Common Toxicities |
---|---|---|---|
PD 0332991 | 185.14 | 111.78 | Neutropenia |
Ribociclib | 286.75 | 196.25 | Neutropenia |
Abemaciclib | 225.68 | 360.68 | Diarrhea, Fatigue |
Case Studies
Several case studies have documented the effectiveness of PD 0332991 in real-world settings. One notable case involved a patient with locally advanced breast cancer who underwent treatment with PD 0332991 combined with letrozole. After six months of therapy, significant tumor reduction was observed without major adverse effects .
Properties
IUPAC Name |
4-[[2-[(1-benzylindol-5-yl)amino]-6-(ethylamino)pyrimidin-4-yl]amino]cyclohexan-1-ol | |
---|---|---|
Source | PubChem | |
URL | https://pubchem.ncbi.nlm.nih.gov | |
Description | Data deposited in or computed by PubChem | |
InChI |
InChI=1S/C27H32N6O/c1-2-28-25-17-26(29-21-8-11-23(34)12-9-21)32-27(31-25)30-22-10-13-24-20(16-22)14-15-33(24)18-19-6-4-3-5-7-19/h3-7,10,13-17,21,23,34H,2,8-9,11-12,18H2,1H3,(H3,28,29,30,31,32) | |
Source | PubChem | |
URL | https://pubchem.ncbi.nlm.nih.gov | |
Description | Data deposited in or computed by PubChem | |
InChI Key |
YVXCDLCJCIDFHE-UHFFFAOYSA-N | |
Source | PubChem | |
URL | https://pubchem.ncbi.nlm.nih.gov | |
Description | Data deposited in or computed by PubChem | |
Canonical SMILES |
CCNC1=CC(=NC(=N1)NC2=CC3=C(C=C2)N(C=C3)CC4=CC=CC=C4)NC5CCC(CC5)O | |
Source | PubChem | |
URL | https://pubchem.ncbi.nlm.nih.gov | |
Description | Data deposited in or computed by PubChem | |
Molecular Formula |
C27H32N6O | |
Source | PubChem | |
URL | https://pubchem.ncbi.nlm.nih.gov | |
Description | Data deposited in or computed by PubChem | |
Molecular Weight |
456.6 g/mol | |
Source | PubChem | |
URL | https://pubchem.ncbi.nlm.nih.gov | |
Description | Data deposited in or computed by PubChem | |
Retrosynthesis Analysis
AI-Powered Synthesis Planning: Our tool employs the Template_relevance Pistachio, Template_relevance Bkms_metabolic, Template_relevance Pistachio_ringbreaker, Template_relevance Reaxys, Template_relevance Reaxys_biocatalysis model, leveraging a vast database of chemical reactions to predict feasible synthetic routes.
One-Step Synthesis Focus: Specifically designed for one-step synthesis, it provides concise and direct routes for your target compounds, streamlining the synthesis process.
Accurate Predictions: Utilizing the extensive PISTACHIO, BKMS_METABOLIC, PISTACHIO_RINGBREAKER, REAXYS, REAXYS_BIOCATALYSIS database, our tool offers high-accuracy predictions, reflecting the latest in chemical research and data.
Strategy Settings
Precursor scoring | Relevance Heuristic |
---|---|
Min. plausibility | 0.01 |
Model | Template_relevance |
Template Set | Pistachio/Bkms_metabolic/Pistachio_ringbreaker/Reaxys/Reaxys_biocatalysis |
Top-N result to add to graph | 6 |
Feasible Synthetic Routes
Disclaimer and Information on In-Vitro Research Products
Please be aware that all articles and product information presented on BenchChem are intended solely for informational purposes. The products available for purchase on BenchChem are specifically designed for in-vitro studies, which are conducted outside of living organisms. In-vitro studies, derived from the Latin term "in glass," involve experiments performed in controlled laboratory settings using cells or tissues. It is important to note that these products are not categorized as medicines or drugs, and they have not received approval from the FDA for the prevention, treatment, or cure of any medical condition, ailment, or disease. We must emphasize that any form of bodily introduction of these products into humans or animals is strictly prohibited by law. It is essential to adhere to these guidelines to ensure compliance with legal and ethical standards in research and experimentation.