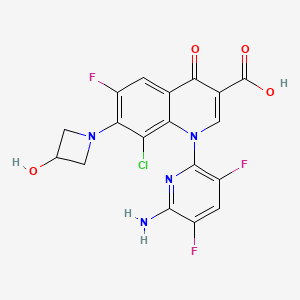
Delafloxacin
Overview
Description
Delafloxacin is a novel anionic fluoroquinolone approved by the U.S. FDA in 2017 for acute bacterial skin and skin structure infections (ABSSSI) . Unlike traditional fluoroquinolones, this compound exhibits enhanced antibacterial activity in acidic environments (e.g., infected tissues with pH ~5.5) due to its unique anionic structure, which facilitates intracellular accumulation . It inhibits both DNA gyrase and topoisomerase IV, reducing the likelihood of resistance development . This compound is available in intravenous (IV) and oral formulations, with broad-spectrum activity against Gram-positive pathogens (including methicillin-resistant Staphylococcus aureus [MRSA]) and Gram-negative bacteria .
Preparation Methods
Synthetic Routes and Reaction Conditions
Delafloxacin is synthesized through a multi-step process involving the condensation of ethoxy acrylic ester with 2,6-diamino-3,5-difluoropyridine. The reaction typically occurs in an organic solvent such as ether, tetrahydrofuran (THF), or methylene dichloride at temperatures ranging from 0 to 100°C . The intermediate products are further processed to yield this compound.
Industrial Production Methods
Industrial production of this compound involves optimizing the synthetic route to ensure high yield and purity. The process includes the use of advanced techniques such as ultra-performance liquid chromatography (UPLC) and mass spectrometry (MS) for quantitation and quality control .
Chemical Reactions Analysis
Metabolic Pathways and Biotransformation
Delafloxacin undergoes phase II metabolism primarily via glucuronidation , mediated by UDP-glucuronosyltransferases (UGTs):
-
UGT1A1 (major)
-
UGT1A3
-
UGT2B15
Approximately 20% of the administered dose is converted to its glucuronide metabolite, which is pharmacologically inactive . Less than 1% undergoes oxidative metabolism, indicating minimal involvement of cytochrome P450 enzymes .
Key Metabolites
Metabolite | Pathway | Excretion Route | Bioactivity |
---|---|---|---|
This compound | Unchanged | Urine (41%), Feces (28%) | Active |
This compound glucuronide | Glucuronidation | Urine (20%), Feces (N/A) | Inactive |
Chelation Reactions
This compound forms stable chelates with multivalent cations , reducing its bioavailability:
-
Cations : Mg²⁺, Al³⁺, Fe²⁺, Zn²⁺
-
Clinical implication : Administration with antacids, iron, or zinc supplements must be separated by ≥2 hours (oral) or ≥6 hours (IV) .
Impact of Chelation on Pharmacokinetics
Parameter | With Chelation | Without Chelation |
---|---|---|
Oral bioavailability | Reduced by 30–50% | 58.8% |
Tₘₐₓ | Delayed | 0.75–4 hours |
Protein Binding and Redox Stability
-
Redox reactivity : No significant oxidation under physiological conditions. The chlorine atom at position C8 and fluorine substituents enhance stability against enzymatic degradation .
pH-Dependent Ionization and Activity
The lack of a protonable group at position C7 results in pH-dependent ionization:
-
Acidic environments (e.g., abscesses) : Increased non-ionized fraction enhances membrane permeability and bactericidal activity .
-
Neutral/basic environments : Higher ionization reduces cellular uptake.
Ionization States Across pH Levels
pH Range | Ionization State | Antibacterial Efficacy |
---|---|---|
5.0–6.0 | Non-ionized | High (MIC₉₀: 0.008–0.5 mg/L) |
7.0–8.0 | Ionized | Moderate (MIC₉₀: 0.06–4 mg/L) |
Resistance-Associated Enzymatic Interactions
Mutations in bacterial DNA gyrase (GyrA Ser83Leu, Asp87Asn) and topoisomerase IV (ParC Ser80Ile, Glu84Val) reduce this compound binding affinity by introducing hydrophobic residues at target sites . These substitutions disrupt hydrogen bonding and electrostatic interactions critical for inhibition.
Impact of Mutations on MIC Values
Mutation(s) | MIC (mg/L) | Fold Increase vs Wild-Type |
---|---|---|
None (Wild-Type) | 0.008 | 1× |
GyrA Ser83Leu | 0.12 | 15× |
GyrA Ser83Leu + ParC Ser80Ile | 1.0 | 125× |
Excretion and Environmental Degradation
-
Urinary excretion : 50–66% (unchanged drug and glucuronide) .
-
Environmental stability : Degrades photolytically in aqueous solutions via defluorination and quinoline-ring cleavage .
Synthetic and Stability Considerations
-
Synthesis : The 4-quinolone core is synthesized through cyclization of a substituted aniline derivative with diethyl ethoxymethylenemalonate, followed by halogenation and azetidine ring introduction .
-
Storage : Stable at 25°C in lyophilized form; reconstituted solutions degrade within 24 hours at room temperature .
This compound’s chemical profile—marked by targeted glucuronidation, cation chelation, and pH-dependent ionization—defines its clinical utility and resistance patterns. Its structural resilience against common bacterial resistance mechanisms positions it as a critical agent for multidrug-resistant infections, though cation interactions and metabolic pathways require careful clinical management.
Scientific Research Applications
Randomized Controlled Trials
Recent studies have demonstrated that delafloxacin is comparable in efficacy to traditional antibiotics. A systematic review of six randomized controlled trials involving over 3,000 patients indicated that this compound had a microbiological eradication rate similar to that of comparator antibiotics (OR = 1.33%, 95% CI = 0.94–1.88) . Moreover, a Phase II trial showed that this compound achieved a higher cure rate compared to vancomycin in treating ABSSSIs, particularly in obese patients .
Safety Profile
This compound is generally well tolerated, with a low incidence of treatment-emergent adverse events (TEAEs). In pooled analyses from Phase III studies, fewer patients experienced adverse events compared to those receiving comparator treatments (7.0% vs. 9.2%) with serious adverse events occurring at similar rates across groups . Notably, no significant differences were observed in the rates of discontinuation due to treatment-related adverse events .
Off-Label Uses and Case Studies
This compound has been utilized off-label for various conditions beyond its approved indications. A case series involving five patients highlighted its effectiveness in treating prosthetic joint infections and acute skin infections, with no documented treatment failures or adverse events reported . The flexibility in its use underscores the need for further investigation into its potential applications.
Comparative In Vitro Activity
In vitro studies have shown that this compound possesses superior potency against certain pathogens compared to other fluoroquinolones. For instance, it demonstrated at least 64-fold greater potency against Staphylococcus aureus than levofloxacin and ciprofloxacin . This enhanced activity against resistant strains positions this compound as a valuable option in the era of increasing antibiotic resistance.
Mechanism of Action
Delafloxacin exerts its antibacterial effects by inhibiting the activity of bacterial DNA topoisomerase IV and DNA gyrase (topoisomerase II). These enzymes are crucial for DNA replication, transcription, and repair. By preventing the relaxation of positive supercoils introduced during DNA elongation, this compound disrupts bacterial DNA processes, leading to cell death .
Comparison with Similar Compounds
Comparative Efficacy
Clinical Response in ABSSSI
Delafloxacin demonstrates superior or comparable efficacy to other antibiotics in treating ABSSSI:
- Composite Clinical Response : Superior to vancomycin, ceftobiprole, fusidic acid, and iclaprim (odds ratio [OR]: 1.05–1.27) .
- Early Clinical Response : Comparable to dalbavancin, daptomycin, linezolid, and vancomycin+aztreonam .
- Microbiological Response : Similar to ceftaroline, linezolid, and vancomycin+aztreonam .
In MRSA-specific populations, this compound’s efficacy aligns with ceftaroline and tigecycline . For obese patients, it matches vancomycin+aztreonam and linezolid .
Community-Acquired Pneumonia (CAP)
This compound shows comparable clinical success to moxifloxacin in hospitalized CAP patients, with a 16-fold greater in vitro activity against Gram-positive pathogens .
Pharmacokinetics (PK) and Dosing
This compound’s PK is linear across doses (50–1600 mg), with minimal accumulation upon repeated dosing . Renal impairment necessitates IV dose adjustments .
Resistance Mechanisms
This compound’s dual enzyme targeting reduces resistance risk compared to older fluoroquinolones . However, resistance in Escherichia coli and Klebsiella pneumoniae is linked to efflux pumps (e.g., AcrAB-TolC), which can be inhibited to restore activity . In contrast, resistance to ciprofloxacin often arises from mutations in gyrA or parC .
Unique Advantages Over Comparators
pH-Dependent Potency : Enhanced activity in acidic infection sites (e.g., abscesses) compared to ciprofloxacin or moxifloxacin .
Dual-Targeting Mechanism : Lowers resistance emergence versus single-target agents like ceftaroline .
Oral/IV Flexibility : Seamless transition between formulations, unlike vancomycin .
Biological Activity
Delafloxacin is a novel anionic fluoroquinolone antibiotic that has garnered attention for its broad-spectrum activity against various bacterial pathogens, including both Gram-positive and Gram-negative organisms. This article explores the biological activity of this compound, focusing on its mechanisms of action, efficacy in clinical studies, and comparative performance against other antibiotics.
This compound exhibits a dual-targeting mechanism, inhibiting both DNA gyrase and topoisomerase IV, which are essential enzymes for bacterial DNA replication and transcription. This dual-targeting capability is attributed to specific substitutions at the C-7 and C-8 positions of the fluoroquinolone nucleus, enhancing its affinity for these targets compared to other fluoroquinolones . The unique molecular structure of this compound allows it to maintain activity against strains exhibiting resistance mechanisms, such as mutations in the quinolone resistance-determining region (QRDR) and efflux pump overexpression .
In Vitro Activity
This compound has demonstrated significant in vitro activity against a range of pathogens. A comparative study found that this compound exhibited at least 64 times greater potency than levofloxacin and ciprofloxacin against Staphylococcus aureus (MIC50 ≤ 0.008 mg/L) and coagulase-negative staphylococci (MIC50 0.06 mg/L). Additionally, it showed superior activity against Pseudomonas aeruginosa (MIC50 0.25 mg/L) compared to other fluoroquinolones .
Pathogen | This compound MIC50 (mg/L) | Levofloxacin MIC50 (mg/L) | Ciprofloxacin MIC50 (mg/L) |
---|---|---|---|
Staphylococcus aureus | ≤0.008 | 0.5 | 1 |
Coagulase-negative Staphylococci | 0.06 | 1 | 1 |
Pseudomonas aeruginosa | 0.25 | 1 | 1 |
Enterobacter cloacae | 0.03 | 0.03 | 0.03 |
Clinical Efficacy
This compound has been evaluated in multiple clinical trials for its efficacy in treating acute bacterial skin and skin structure infections (ABSSSIs). In a Phase 3 study involving 850 adults, this compound was compared with vancomycin plus aztreonam. The results showed that this compound achieved an objective response rate of 83.7%, comparable to the vancomycin/aztreonam group at 80.6% . Notably, bacterial eradication rates for MRSA were reported at 100% for this compound-treated patients .
Case Studies
A case series involving five patients treated with this compound for various infections demonstrated high success rates without adverse events. The conditions included prosthetic joint infections and acute skin infections, highlighting this compound's potential off-label applications .
Resistance Mechanisms
Despite its broad-spectrum activity, some resistance mechanisms have been identified. This compound retains efficacy against certain resistant strains due to its unique pharmacological properties. For instance, it has shown effectiveness against levofloxacin-nonsusceptible Staphylococcus aureus isolates, with eradication rates exceeding 98% .
Q & A
Basic Research Questions
Q. What methodologies are recommended for determining Delafloxacin's minimum inhibitory concentration (MIC) in vitro, and how do physiological conditions influence its activity?
this compound's MIC is typically measured under neutral pH conditions using standardized broth microdilution methods. However, its activity is enhanced in acidic environments (e.g., phagosomes), where its anionic structure promotes intracellular accumulation. Researchers should compare MIC values under neutral vs. acidic pH to better predict in vivo efficacy, as standard MICs may underestimate potency in acidic infection sites .
Q. How should stability-indicating analytical methods be validated for this compound in pharmaceutical formulations?
A validated LC-MS/MS method can quantify this compound and its degradation products under stress conditions (e.g., heat, acid/alkali, oxidation). Parameters like linearity (0.01–10 mg/L in plasma), precision (RSD <15%), and recovery rates must adhere to FDA guidelines. Degradation products are characterized using MS/MS in positive ionization mode to ensure specificity .
Q. What pharmacokinetic (PK) properties of this compound are critical for experimental design in animal or human studies?
Key PK considerations include its high tissue penetration, concentration-dependent bactericidal activity, and dual targeting of DNA gyrase/topoisomerase IV. This compound's anionic nature enhances accumulation in acidic sites (e.g., abscesses), requiring PK/PD models to incorporate pH-adjusted MIC values. Oral and IV bioavailability studies should account for food effects, with dose proportionality established up to 1,200 mg .
Advanced Research Questions
Q. How can researchers resolve discrepancies between in vitro MIC data and in vivo efficacy for this compound against gram-negative pathogens?
While in vitro MICs for gram-negative bacteria like E. coli may appear high (e.g., MIC90 = 4 mg/L), this compound's in vivo efficacy is influenced by intracellular accumulation and pH-dependent potency. Animal models simulating infected tissue microenvironments (e.g., murine thigh infection at pH 6.0) are recommended to bridge this gap .
Q. What experimental approaches optimize this compound dosing in obese populations?
Subgroup analyses of RCTs suggest BMI impacts vancomycin efficacy but not this compound, likely due to its consistent tissue distribution. Dosing studies should use population PK modeling to assess AUC/MIC ratios across BMI categories, ensuring therapeutic exposure without dose adjustments .
Q. What mechanisms underlie this compound resistance in MRSA, and how can efflux pump activity be quantified?
Resistance in MRSA arises via mutations in gyrA/parC or genomic amplifications of the sdrM efflux pump gene. Experimental evolution under this compound pressure (≥30 passages) selects for sdrM-amplified strains. Quantify efflux activity using ethidium bromide accumulation assays with/without efflux inhibitors (e.g., reserpine) .
Q. How should meta-analyses account for heterogeneity when comparing this compound's clinical cure rates in ABSSSI trials?
Pool data using random-effects models to handle variability in RCT designs (e.g., comparator drugs, dosing). Stratify by infection type (cellulitis vs. abscess) and pathogen (MRSA vs. MSSA). Sensitivity analyses exclude high-bias studies, focusing on double-blind trials with ITT populations .
Q. What in vitro models best replicate this compound's activity against intracellular pathogens like Mycobacterium tuberculosis?
Use macrophage infection models (e.g., THP-1 cells) incubated at pH 5.5–6.0 to mimic phagosomal acidity. Compare intracellular vs. extracellular MICs, as this compound’s potency increases 10-fold in acidic conditions, enhancing bacterial clearance in M. tuberculosis-infected macrophages .
Q. How do gyrA/parC mutations and efflux pump gene variations impact microbiological eradication rates in clinical trials?
Mutations in gyrA (Ser91Tyr) and parC reduce target affinity, while sdrM amplifications decrease intracellular drug concentrations. In trials, correlate eradication failure with baseline pathogen genotypes using whole-genome sequencing. Presumed eradication (based on clinical cure) may mask resistance, necessitating post-treatment susceptibility testing .
Q. What bioanalytical challenges arise when quantifying this compound in biological matrices, and how are they mitigated?
Matrix effects (e.g., plasma proteins) can lower recovery rates. Use deuterated internal standards (e.g., D5-Delafloxacin) and solid-phase extraction to enhance sensitivity. Validate methods per FDA guidelines, ensuring LLOQ ≤0.01 mg/L in plasma and ≤0.05 mg/L in urine for pharmacokinetic studies .
Q. Tables for Key Data Comparisons
Table 1: this compound MIC90 Values Under Different Conditions
Pathogen | Neutral pH MIC90 (mg/L) | Acidic pH MIC90 (mg/L) | Source |
---|---|---|---|
S. aureus (MRSA) | 0.25 | 0.03 | |
N. gonorrhoeae | 0.125 | N/A | |
E. coli | 4.0 | 0.5 |
Table 2: Clinical Cure Rates in ABSSSI RCTs
Study ID | This compound Cure Rate (%) | Comparator Cure Rate (%) | Odds Ratio (95% CI) |
---|---|---|---|
RCT 1 | 88.5 | 87.1 | 1.12 (0.71–1.77) |
RCT 2 | 89.2 | 90.1 | 0.94 (0.62–1.41) |
Properties
IUPAC Name |
1-(6-amino-3,5-difluoropyridin-2-yl)-8-chloro-6-fluoro-7-(3-hydroxyazetidin-1-yl)-4-oxoquinoline-3-carboxylic acid | |
---|---|---|
Source | PubChem | |
URL | https://pubchem.ncbi.nlm.nih.gov | |
Description | Data deposited in or computed by PubChem | |
InChI |
InChI=1S/C18H12ClF3N4O4/c19-12-13-7(1-9(20)14(12)25-3-6(27)4-25)15(28)8(18(29)30)5-26(13)17-11(22)2-10(21)16(23)24-17/h1-2,5-6,27H,3-4H2,(H2,23,24)(H,29,30) | |
Source | PubChem | |
URL | https://pubchem.ncbi.nlm.nih.gov | |
Description | Data deposited in or computed by PubChem | |
InChI Key |
DYDCPNMLZGFQTM-UHFFFAOYSA-N | |
Source | PubChem | |
URL | https://pubchem.ncbi.nlm.nih.gov | |
Description | Data deposited in or computed by PubChem | |
Canonical SMILES |
C1C(CN1C2=C(C=C3C(=C2Cl)N(C=C(C3=O)C(=O)O)C4=C(C=C(C(=N4)N)F)F)F)O | |
Source | PubChem | |
URL | https://pubchem.ncbi.nlm.nih.gov | |
Description | Data deposited in or computed by PubChem | |
Molecular Formula |
C18H12ClF3N4O4 | |
Source | PubChem | |
URL | https://pubchem.ncbi.nlm.nih.gov | |
Description | Data deposited in or computed by PubChem | |
DSSTOX Substance ID |
DTXSID40172331 | |
Record name | Delafloxacin | |
Source | EPA DSSTox | |
URL | https://comptox.epa.gov/dashboard/DTXSID40172331 | |
Description | DSSTox provides a high quality public chemistry resource for supporting improved predictive toxicology. | |
Molecular Weight |
440.8 g/mol | |
Source | PubChem | |
URL | https://pubchem.ncbi.nlm.nih.gov | |
Description | Data deposited in or computed by PubChem | |
Mechanism of Action |
Delafloxacin inhibits the activity of bacterial DNA topoisomerase IV and DNA gyrase (topoisomerase II). This interferes with bacterial DNA replication by preventing the relaxation of positive supercoils introduced as part of the elongation process. The resultant strain inhibits further elongation. Delafloxacin exerts concentration-dependent bacteriocidal activity. | |
Record name | Delafloxacin | |
Source | DrugBank | |
URL | https://www.drugbank.ca/drugs/DB11943 | |
Description | The DrugBank database is a unique bioinformatics and cheminformatics resource that combines detailed drug (i.e. chemical, pharmacological and pharmaceutical) data with comprehensive drug target (i.e. sequence, structure, and pathway) information. | |
Explanation | Creative Common's Attribution-NonCommercial 4.0 International License (http://creativecommons.org/licenses/by-nc/4.0/legalcode) | |
CAS No. |
189279-58-1 | |
Record name | Delafloxacin | |
Source | CAS Common Chemistry | |
URL | https://commonchemistry.cas.org/detail?cas_rn=189279-58-1 | |
Description | CAS Common Chemistry is an open community resource for accessing chemical information. Nearly 500,000 chemical substances from CAS REGISTRY cover areas of community interest, including common and frequently regulated chemicals, and those relevant to high school and undergraduate chemistry classes. This chemical information, curated by our expert scientists, is provided in alignment with our mission as a division of the American Chemical Society. | |
Explanation | The data from CAS Common Chemistry is provided under a CC-BY-NC 4.0 license, unless otherwise stated. | |
Record name | Delafloxacin [USAN:INN] | |
Source | ChemIDplus | |
URL | https://pubchem.ncbi.nlm.nih.gov/substance/?source=chemidplus&sourceid=0189279581 | |
Description | ChemIDplus is a free, web search system that provides access to the structure and nomenclature authority files used for the identification of chemical substances cited in National Library of Medicine (NLM) databases, including the TOXNET system. | |
Record name | Delafloxacin | |
Source | DrugBank | |
URL | https://www.drugbank.ca/drugs/DB11943 | |
Description | The DrugBank database is a unique bioinformatics and cheminformatics resource that combines detailed drug (i.e. chemical, pharmacological and pharmaceutical) data with comprehensive drug target (i.e. sequence, structure, and pathway) information. | |
Explanation | Creative Common's Attribution-NonCommercial 4.0 International License (http://creativecommons.org/licenses/by-nc/4.0/legalcode) | |
Record name | Delafloxacin | |
Source | EPA DSSTox | |
URL | https://comptox.epa.gov/dashboard/DTXSID40172331 | |
Description | DSSTox provides a high quality public chemistry resource for supporting improved predictive toxicology. | |
Record name | DELAFLOXACIN | |
Source | FDA Global Substance Registration System (GSRS) | |
URL | https://gsrs.ncats.nih.gov/ginas/app/beta/substances/6315412YVF | |
Description | The FDA Global Substance Registration System (GSRS) enables the efficient and accurate exchange of information on what substances are in regulated products. Instead of relying on names, which vary across regulatory domains, countries, and regions, the GSRS knowledge base makes it possible for substances to be defined by standardized, scientific descriptions. | |
Explanation | Unless otherwise noted, the contents of the FDA website (www.fda.gov), both text and graphics, are not copyrighted. They are in the public domain and may be republished, reprinted and otherwise used freely by anyone without the need to obtain permission from FDA. Credit to the U.S. Food and Drug Administration as the source is appreciated but not required. | |
Synthesis routes and methods I
Procedure details
Synthesis routes and methods II
Procedure details
Retrosynthesis Analysis
AI-Powered Synthesis Planning: Our tool employs the Template_relevance Pistachio, Template_relevance Bkms_metabolic, Template_relevance Pistachio_ringbreaker, Template_relevance Reaxys, Template_relevance Reaxys_biocatalysis model, leveraging a vast database of chemical reactions to predict feasible synthetic routes.
One-Step Synthesis Focus: Specifically designed for one-step synthesis, it provides concise and direct routes for your target compounds, streamlining the synthesis process.
Accurate Predictions: Utilizing the extensive PISTACHIO, BKMS_METABOLIC, PISTACHIO_RINGBREAKER, REAXYS, REAXYS_BIOCATALYSIS database, our tool offers high-accuracy predictions, reflecting the latest in chemical research and data.
Strategy Settings
Precursor scoring | Relevance Heuristic |
---|---|
Min. plausibility | 0.01 |
Model | Template_relevance |
Template Set | Pistachio/Bkms_metabolic/Pistachio_ringbreaker/Reaxys/Reaxys_biocatalysis |
Top-N result to add to graph | 6 |
Feasible Synthetic Routes
Disclaimer and Information on In-Vitro Research Products
Please be aware that all articles and product information presented on BenchChem are intended solely for informational purposes. The products available for purchase on BenchChem are specifically designed for in-vitro studies, which are conducted outside of living organisms. In-vitro studies, derived from the Latin term "in glass," involve experiments performed in controlled laboratory settings using cells or tissues. It is important to note that these products are not categorized as medicines or drugs, and they have not received approval from the FDA for the prevention, treatment, or cure of any medical condition, ailment, or disease. We must emphasize that any form of bodily introduction of these products into humans or animals is strictly prohibited by law. It is essential to adhere to these guidelines to ensure compliance with legal and ethical standards in research and experimentation.