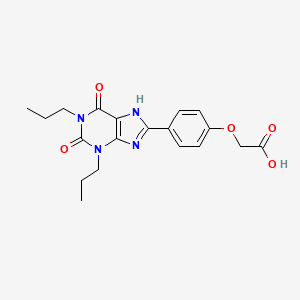
XCC
Overview
Description
Xanthomonas citri subsp. citri (XCC) is a bacterium known for causing citrus canker, a severe disease affecting citrus plants. This pathogen is responsible for significant economic losses in citrus production worldwide. Xanthomonas citri subsp. citri infects various citrus species, leading to lesions on leaves, stems, and fruits, which can result in premature fruit drop and reduced yield .
Preparation Methods
The preparation of Xanthomonas citri subsp. citri involves culturing the bacterium under specific conditions. The bacterium is typically isolated from infected citrus tissues and grown on nutrient-rich media. Industrial production methods for Xanthomonas citri subsp. citri include large-scale fermentation processes where the bacterium is cultured in bioreactors under controlled conditions to produce sufficient quantities for research and agricultural applications .
Chemical Reactions Analysis
Xanthomonas citri subsp. citri undergoes various chemical reactions, including:
Oxidation: The bacterium can oxidize certain organic compounds, contributing to its pathogenicity.
Reduction: Reduction reactions are involved in the bacterium’s metabolic processes.
Substitution: Xanthomonas citri subsp. citri can participate in substitution reactions, particularly in its interactions with plant tissues. Common reagents used in these reactions include various organic substrates and enzymes produced by the bacterium. .
Scientific Research Applications
Xanthomonas citri subsp. citri has several scientific research applications:
Chemistry: Studying the bacterium’s metabolic pathways and chemical interactions with host plants.
Biology: Understanding the mechanisms of bacterial infection and plant defense responses.
Medicine: Exploring potential antibacterial compounds that can inhibit Xanthomonas citri subsp. citri.
Industry: Developing biocontrol agents and strategies to manage citrus canker in agricultural settings
Mechanism of Action
The mechanism of action of Xanthomonas citri subsp. citri involves several molecular targets and pathways:
Infection Process: The bacterium enters the plant through stomata or wounds and colonizes the intercellular spaces.
Virulence Factors: Xanthomonas citri subsp. citri produces virulence factors such as extracellular polysaccharides and proteins that facilitate infection and disease progression.
Host Interaction: The bacterium interacts with host plant cells, leading to the formation of lesions and cankers
Comparison with Similar Compounds
Xanthomonas citri subsp. citri can be compared with other similar phytopathogenic bacteria, such as:
Properties
IUPAC Name |
2-[4-(2,6-dioxo-1,3-dipropyl-7H-purin-8-yl)phenoxy]acetic acid | |
---|---|---|
Source | PubChem | |
URL | https://pubchem.ncbi.nlm.nih.gov | |
Description | Data deposited in or computed by PubChem | |
InChI |
InChI=1S/C19H22N4O5/c1-3-9-22-17-15(18(26)23(10-4-2)19(22)27)20-16(21-17)12-5-7-13(8-6-12)28-11-14(24)25/h5-8H,3-4,9-11H2,1-2H3,(H,20,21)(H,24,25) | |
Source | PubChem | |
URL | https://pubchem.ncbi.nlm.nih.gov | |
Description | Data deposited in or computed by PubChem | |
InChI Key |
QTMMGCYGCFXBFI-UHFFFAOYSA-N | |
Source | PubChem | |
URL | https://pubchem.ncbi.nlm.nih.gov | |
Description | Data deposited in or computed by PubChem | |
Canonical SMILES |
CCCN1C2=C(C(=O)N(C1=O)CCC)NC(=N2)C3=CC=C(C=C3)OCC(=O)O | |
Source | PubChem | |
URL | https://pubchem.ncbi.nlm.nih.gov | |
Description | Data deposited in or computed by PubChem | |
Molecular Formula |
C19H22N4O5 | |
Source | PubChem | |
URL | https://pubchem.ncbi.nlm.nih.gov | |
Description | Data deposited in or computed by PubChem | |
DSSTOX Substance ID |
DTXSID20914271 | |
Record name | [4-(2,6-Dioxo-1,3-dipropyl-2,3,6,7-tetrahydro-1H-purin-8-yl)phenoxy]acetic acid | |
Source | EPA DSSTox | |
URL | https://comptox.epa.gov/dashboard/DTXSID20914271 | |
Description | DSSTox provides a high quality public chemistry resource for supporting improved predictive toxicology. | |
Molecular Weight |
386.4 g/mol | |
Source | PubChem | |
URL | https://pubchem.ncbi.nlm.nih.gov | |
Description | Data deposited in or computed by PubChem | |
CAS No. |
96865-83-7 | |
Record name | 8-(4-Carboxymethyloxy)phenyl-1,3-dipropylxanthine | |
Source | ChemIDplus | |
URL | https://pubchem.ncbi.nlm.nih.gov/substance/?source=chemidplus&sourceid=0096865837 | |
Description | ChemIDplus is a free, web search system that provides access to the structure and nomenclature authority files used for the identification of chemical substances cited in National Library of Medicine (NLM) databases, including the TOXNET system. | |
Record name | [4-(2,6-Dioxo-1,3-dipropyl-2,3,6,7-tetrahydro-1H-purin-8-yl)phenoxy]acetic acid | |
Source | EPA DSSTox | |
URL | https://comptox.epa.gov/dashboard/DTXSID20914271 | |
Description | DSSTox provides a high quality public chemistry resource for supporting improved predictive toxicology. | |
Retrosynthesis Analysis
AI-Powered Synthesis Planning: Our tool employs the Template_relevance Pistachio, Template_relevance Bkms_metabolic, Template_relevance Pistachio_ringbreaker, Template_relevance Reaxys, Template_relevance Reaxys_biocatalysis model, leveraging a vast database of chemical reactions to predict feasible synthetic routes.
One-Step Synthesis Focus: Specifically designed for one-step synthesis, it provides concise and direct routes for your target compounds, streamlining the synthesis process.
Accurate Predictions: Utilizing the extensive PISTACHIO, BKMS_METABOLIC, PISTACHIO_RINGBREAKER, REAXYS, REAXYS_BIOCATALYSIS database, our tool offers high-accuracy predictions, reflecting the latest in chemical research and data.
Strategy Settings
Precursor scoring | Relevance Heuristic |
---|---|
Min. plausibility | 0.01 |
Model | Template_relevance |
Template Set | Pistachio/Bkms_metabolic/Pistachio_ringbreaker/Reaxys/Reaxys_biocatalysis |
Top-N result to add to graph | 6 |
Feasible Synthetic Routes
- Type III secretion system (T3SS) and effectors: Xcc utilizes T3SS to deliver effector proteins (T3Es) directly into host cells, interfering with plant defense mechanisms and promoting disease [, , , , ].
- Genetic diversity and pathogenicity: Different this compound races exhibit varying degrees of virulence on different host cultivars, suggesting a complex interplay of pathogenicity factors and host resistance genes [, , , , ].
- Molecular mechanisms of pathogenicity: Studies investigate the role of specific genes and proteins in this compound virulence, including those involved in xanthan production, extracellular enzyme secretion, and quorum sensing [, , , , , , , , , , ].
- Host responses to this compound infection: Research explores plant defense mechanisms against this compound, including stomatal aperture regulation, callose deposition, and the role of specific resistance genes [, , , ].
- Control and management of black rot: Studies evaluate various approaches to manage this compound, such as copper-based treatments, biocontrol agents, and breeding for resistance [, , , ].
Q1: What is the role of the Type III secretion system (T3SS) in this compound virulence?
A1: The T3SS is a needle-like apparatus used by this compound to inject effector proteins (T3Es) directly into host plant cells. These effectors suppress plant immunity and manipulate host cellular processes to the benefit of the bacteria, ultimately contributing to disease development [, , , , ].
Q2: How do different races of this compound contribute to variations in disease severity?
A2: this compound exists as different races, each carrying a unique repertoire of virulence factors, including T3Es. These variations contribute to differences in their ability to infect specific host cultivars and cause disease. Some races are more aggressive than others, leading to a wider range of susceptible hosts and more severe symptoms [, , , , ].
Q3: How does xanthan production contribute to this compound virulence?
A3: Xanthan is an exopolysaccharide produced by this compound. It plays a crucial role in virulence by forming a protective biofilm around bacterial cells, contributing to clogging of the plant vascular system, and hindering plant defenses [, ].
Q4: How does this compound evade recognition by the host immune system?
A4: this compound employs various strategies to evade host recognition. One mechanism involves variations in the composition of exopolysaccharides (EPS) and lipopolysaccharides (LPS), which can help the bacteria avoid detection by the plant's immune system [].
Q5: How do plants defend themselves against this compound infection?
A5: Plants employ various defense mechanisms against this compound, including stomatal closure to prevent bacterial entry, callose deposition to reinforce cell walls and limit bacterial spread, and the activation of specific resistance genes that trigger immune responses [, , , ].
Q6: Why are some Brassica varieties more susceptible to black rot than others?
A6: Resistance or susceptibility to this compound is often determined by a gene-for-gene interaction between the pathogen's effector repertoire and the host's resistance genes. Variations in these genes among Brassica varieties contribute to differences in their ability to recognize and defend against this compound infection [, , , ].
Q7: What are some effective ways to manage black rot disease caused by this compound?
A7: Management strategies include: * Cultural practices: Crop rotation, use of disease-free seeds and transplants, and sanitation to reduce inoculum levels [, ].* Chemical control: Copper-based bactericides can be effective, but resistance development is a concern [, , ].* Biological control: Utilizing beneficial microorganisms like Bacillus subtilis to suppress this compound growth []. * Host resistance: Breeding resistant cultivars is the most sustainable approach to manage black rot [, , , ].
Disclaimer and Information on In-Vitro Research Products
Please be aware that all articles and product information presented on BenchChem are intended solely for informational purposes. The products available for purchase on BenchChem are specifically designed for in-vitro studies, which are conducted outside of living organisms. In-vitro studies, derived from the Latin term "in glass," involve experiments performed in controlled laboratory settings using cells or tissues. It is important to note that these products are not categorized as medicines or drugs, and they have not received approval from the FDA for the prevention, treatment, or cure of any medical condition, ailment, or disease. We must emphasize that any form of bodily introduction of these products into humans or animals is strictly prohibited by law. It is essential to adhere to these guidelines to ensure compliance with legal and ethical standards in research and experimentation.