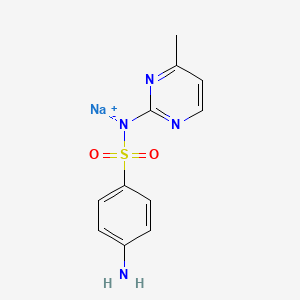
Sulfamerazine sodium
Overview
Description
Mechanism of Action
Target of Action
Sulfamerazine sodium primarily targets the bacterial enzyme dihydropteroate synthetase . This enzyme plays a crucial role in the synthesis of dihydrofolic acid, a precursor to folic acid, which is essential for bacterial growth and reproduction .
Mode of Action
This compound acts by inhibiting the bacterial synthesis of dihydrofolic acid . It achieves this by competing with para-aminobenzoic acid (PABA) for binding to dihydropteroate synthetase . By blocking this binding, this compound prevents the formation of dihydrofolic acid, thereby inhibiting bacterial growth . This makes this compound a bacteriostatic agent .
Biochemical Pathways
The primary biochemical pathway affected by this compound is the synthesis of folic acid in bacteria . By inhibiting the enzyme dihydropteroate synthetase, this compound disrupts the conversion of PABA to dihydrofolic acid . This interruption in the folic acid synthesis pathway leads to a deficiency of this essential vitamin in the bacteria, inhibiting their growth and reproduction .
Pharmacokinetics
Sulfonamides, the class of drugs to which this compound belongs, are generally well absorbed from the gastrointestinal tract, widely distributed throughout all tissues, metabolized in the liver, and excreted in the urine .
Result of Action
The primary result of this compound’s action is the inhibition of bacterial growth . By preventing the synthesis of dihydrofolic acid, an essential component for bacterial growth and reproduction, this compound effectively halts the proliferation of the bacteria .
Action Environment
The action of this compound can be influenced by various environmental factors. For instance, the presence of pus can inhibit the antibacterial action of sulfonamides . Additionally, the interaction of this compound with other substances, such as cyclodextrins, can influence its diffusion and potentially reduce its toxicity .
Biochemical Analysis
Biochemical Properties
Sulfamerazine sodium inhibits bacterial synthesis of dihydrofolic acid by competing with para-aminobenzoic acid (PABA) for binding to dihydropteroate synthetase . This interaction is crucial in the biochemical reactions that the drug participates in. The inhibition of dihydrofolic acid synthesis decreases the synthesis of bacterial nucleotides and DNA .
Cellular Effects
This compound exerts its effects on various types of cells, primarily bacterial cells. By inhibiting the synthesis of dihydrofolic acid, it disrupts bacterial DNA synthesis, thereby inhibiting bacterial growth . This can influence cell function, including impacts on cell signaling pathways, gene expression, and cellular metabolism .
Molecular Mechanism
The molecular mechanism of action of this compound involves its competition with PABA for binding to dihydropteroate synthetase . This competition inhibits the synthesis of dihydrofolic acid, a precursor to tetrahydrofolic acid, which is necessary for the synthesis of purines and dTMP . This inhibition disrupts bacterial DNA synthesis and results in bacteriostatic effects .
Dosage Effects in Animal Models
In animal models, the effects of this compound can vary with different dosages
Metabolic Pathways
This compound is involved in the metabolic pathway that synthesizes dihydrofolic acid in bacteria . It competes with PABA for binding to dihydropteroate synthetase, an enzyme involved in the synthesis of dihydrofolic acid .
Transport and Distribution
As a sulfonamide, it is likely to be distributed throughout the body after administration .
Subcellular Localization
Given its mechanism of action, it is likely to be found in the cytoplasm where it can interact with dihydropteroate synthetase .
Preparation Methods
Synthetic Routes and Reaction Conditions
Sulfamerazine sodium is synthesized through a series of chemical reactions involving sulfanilamide and other reagents. The synthesis typically involves the following steps:
Nitration: Sulfanilamide is nitrated to form a nitro derivative.
Reduction: The nitro derivative is reduced to form an amino derivative.
Condensation: The amino derivative is condensed with a suitable aldehyde or ketone to form the final product.
Industrial Production Methods
In industrial settings, the production of this compound involves large-scale chemical reactors and stringent quality control measures to ensure the purity and efficacy of the final product. The process includes:
Raw Material Preparation: High-purity sulfanilamide and other reagents are prepared.
Reaction Control: The reactions are carefully monitored to maintain optimal conditions.
Purification: The final product is purified using techniques such as crystallization and filtration.
Chemical Reactions Analysis
Types of Reactions
Sulfamerazine sodium undergoes various chemical reactions, including:
Oxidation: It can be oxidized to form sulfonic acid derivatives.
Reduction: It can be reduced to form amine derivatives.
Substitution: It can undergo substitution reactions with various nucleophiles.
Common Reagents and Conditions
Oxidation: Common oxidizing agents include potassium permanganate and hydrogen peroxide.
Reduction: Common reducing agents include sodium borohydride and lithium aluminum hydride.
Substitution: Common nucleophiles include halides and amines.
Major Products Formed
Oxidation: Sulfonic acid derivatives.
Reduction: Amine derivatives.
Substitution: Various substituted sulfonamide derivatives.
Scientific Research Applications
Sulfamerazine sodium has a wide range of scientific research applications, including:
Chemistry: Used as a model compound in studies of sulfonamide chemistry and reactivity.
Biology: Used in studies of bacterial resistance mechanisms and the development of new antibacterial agents.
Medicine: Used in clinical research to evaluate its efficacy and safety in treating bacterial infections.
Industry: Used in the development of new pharmaceutical formulations and drug delivery systems
Comparison with Similar Compounds
Similar Compounds
Sulfadiazine: Another sulfonamide antibacterial agent used in combination with pyrimethamine to treat toxoplasmosis.
Sulfamethazine: Used to treat bacterial infections in veterinary medicine.
Uniqueness
Sulfamerazine sodium is unique in its specific structure, which includes a methyl group on the pyrimidine ring. This structural feature contributes to its distinct pharmacokinetic properties and spectrum of antibacterial activity .
Biological Activity
Sulfamerazine sodium, a long-acting sulfanilamide antibacterial agent, is a sodium salt form of sulfamerazine. This compound exhibits a range of biological activities, particularly as an antimicrobial agent. This article explores the biological activity of this compound, including its pharmacological mechanisms, clinical applications, and research findings.
This compound functions primarily by inhibiting bacterial folic acid synthesis. It competes with para-aminobenzoic acid (PABA) for the active site of dihydropteroate synthase, an enzyme critical for the synthesis of dihydrofolate, which is necessary for nucleic acid synthesis. This inhibition ultimately disrupts bacterial growth and replication, making it effective against various bacterial infections.
Antimicrobial Activity
This compound has demonstrated effectiveness against both Gram-positive and Gram-negative bacteria. A study evaluated its antimicrobial properties against several strains, including:
- Gram-positive bacteria : Staphylococcus aureus, Enterococcus faecalis
- Gram-negative bacteria : Pseudomonas aeruginosa, Escherichia coli
- Fungi : Candida albicans
The results indicated that this compound exhibited significant antimicrobial activity, particularly when used in combination with metal complexes like Ru(III), which enhanced its efficacy by improving cellular penetration and blocking metal-binding sites in microbial enzymes .
Clinical Applications
This compound has been utilized in various clinical settings. A clinical evaluation involving 116 patients highlighted its therapeutic potential while noting some renal complications associated with its use. The study emphasized the need for careful monitoring due to possible adverse effects .
Table 1: Clinical Evaluation Summary
Parameter | Value |
---|---|
Number of Patients | 116 |
Common Side Effects | Renal complications |
Efficacy | High against bacterial infections |
Toxicity and Safety Profile
Research has indicated that while this compound is effective as an antimicrobial agent, it also poses certain toxicity risks. For instance, a study on its interaction with cyclodextrins suggested that these compounds could mitigate some adverse effects of sulfamerazine, potentially enhancing its therapeutic profile .
Case Studies and Research Findings
- Antimicrobial Efficacy Enhancement : A study demonstrated that the combination of sulfamerazine with Ru(III) complexes significantly increased its antimicrobial activity compared to sulfamerazine alone. The IC50 values were determined to be 183 µM and 187 µM for cancer cell lines MCF-7 and PC3 respectively .
- Toxicity Mitigation via Cyclodextrins : In experiments involving pollen germination and BY-2 cell tests, the presence of β-cyclodextrin reduced the toxicity of this compound, indicating potential for safer formulations .
- Pharmacokinetics : A diffusion study revealed that this compound has low solubility in water (<0.8 mM), which can affect its bioavailability. The study provided diffusion coefficients under varying concentrations to better understand its behavior in biological systems .
Table 2: Diffusion Coefficients of this compound
Concentration (mol dm) | Diffusion Coefficient (×10 ms) |
---|---|
0.000 | 0.944 ± 0.003 |
0.005 | 0.927 ± 0.005 |
0.010 | 0.906 ± 0.003 |
Properties
CAS No. |
127-58-2 |
---|---|
Molecular Formula |
C11H12N4NaO2S |
Molecular Weight |
287.30 g/mol |
IUPAC Name |
sodium;(4-aminophenyl)sulfonyl-(4-methylpyrimidin-2-yl)azanide |
InChI |
InChI=1S/C11H12N4O2S.Na/c1-8-6-7-13-11(14-8)15-18(16,17)10-4-2-9(12)3-5-10;/h2-7H,12H2,1H3,(H,13,14,15); |
InChI Key |
XEAKUBSUGWQDRM-UHFFFAOYSA-N |
SMILES |
CC1=NC(=NC=C1)[N-]S(=O)(=O)C2=CC=C(C=C2)N.[Na+] |
Canonical SMILES |
CC1=NC(=NC=C1)NS(=O)(=O)C2=CC=C(C=C2)N.[Na] |
Appearance |
White or light yellow crystalline powder |
Key on ui other cas no. |
127-58-2 |
Pictograms |
Irritant |
Synonyms |
sodium; (4-aminophenyl)sulfonyl-(4-methylpyrimidin-2-yl)azanide |
Origin of Product |
United States |
Retrosynthesis Analysis
AI-Powered Synthesis Planning: Our tool employs the Template_relevance Pistachio, Template_relevance Bkms_metabolic, Template_relevance Pistachio_ringbreaker, Template_relevance Reaxys, Template_relevance Reaxys_biocatalysis model, leveraging a vast database of chemical reactions to predict feasible synthetic routes.
One-Step Synthesis Focus: Specifically designed for one-step synthesis, it provides concise and direct routes for your target compounds, streamlining the synthesis process.
Accurate Predictions: Utilizing the extensive PISTACHIO, BKMS_METABOLIC, PISTACHIO_RINGBREAKER, REAXYS, REAXYS_BIOCATALYSIS database, our tool offers high-accuracy predictions, reflecting the latest in chemical research and data.
Strategy Settings
Precursor scoring | Relevance Heuristic |
---|---|
Min. plausibility | 0.01 |
Model | Template_relevance |
Template Set | Pistachio/Bkms_metabolic/Pistachio_ringbreaker/Reaxys/Reaxys_biocatalysis |
Top-N result to add to graph | 6 |
Feasible Synthetic Routes
Disclaimer and Information on In-Vitro Research Products
Please be aware that all articles and product information presented on BenchChem are intended solely for informational purposes. The products available for purchase on BenchChem are specifically designed for in-vitro studies, which are conducted outside of living organisms. In-vitro studies, derived from the Latin term "in glass," involve experiments performed in controlled laboratory settings using cells or tissues. It is important to note that these products are not categorized as medicines or drugs, and they have not received approval from the FDA for the prevention, treatment, or cure of any medical condition, ailment, or disease. We must emphasize that any form of bodily introduction of these products into humans or animals is strictly prohibited by law. It is essential to adhere to these guidelines to ensure compliance with legal and ethical standards in research and experimentation.