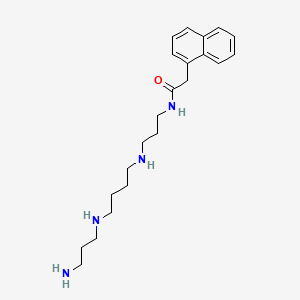
Naspm
Overview
Description
1-Naphthylacetyl spermine, commonly referred to as Naspm, is a synthetic analogue of Joro spider toxin. It is known for its role as a calcium-permeable AMPA receptor antagonist. This compound is particularly significant in neuroscience research due to its ability to selectively suppress inwardly rectifying and calcium-permeable AMPA receptors expressed in type II neurons .
Mechanism of Action
Target of Action
NASPM, also known as 1-Naphthylacetylspermine, is a synthetic analogue of Joro spider toxin . Its primary targets are the calcium-permeable AMPA receptors (CP-AMPARs) . These receptors are a type of ionotropic transmembrane receptor for glutamate that mediates fast synaptic transmission in the central nervous system .
Biochemical Pathways
This compound’s action on CP-AMPARs affects the biochemical pathways involving these receptors. The suppression of CP-AMPARs can influence synaptic plasticity and pathology, as these receptors contribute to many forms of these processes .
Pharmacokinetics
It’s known that this compound can be applied intracellularly , suggesting it can cross cell membranes.
Result of Action
The result of this compound’s action is the complete block of GluA1-mediated outward currents, irrespective of the type of associated auxiliary subunit . In neurons from GluA2-knockout mice expressing only CP-AMPARs, intracellular this compound, unlike spermine, completely blocks outward synaptic currents .
Action Environment
It’s known that the blocking effect of this compound on the calcium-permeable ampa receptors is use and voltage-dependent , suggesting that the electrical environment of the neuron can influence its action.
Biochemical Analysis
Biochemical Properties
1-Naphthylacetylspermine interacts with AMPA receptors, specifically the calcium-permeable AMPA receptors . It acts as an antagonist, blocking the activity of these receptors . This interaction is crucial in the regulation of calcium influx into the neurons, which plays a significant role in neuronal signaling and function .
Cellular Effects
The effects of 1-Naphthylacetylspermine on cells are primarily related to its interaction with AMPA receptors. By blocking these receptors, 1-Naphthylacetylspermine can influence cell function, including impacts on cell signaling pathways and cellular metabolism . For instance, it has been used to study the involvement of AMPA receptors in the biphasic activation of stress-activated protein kinase/extracellular signal-regulated kinase-1 .
Molecular Mechanism
At the molecular level, 1-Naphthylacetylspermine exerts its effects by binding to AMPA receptors and blocking their activity . This binding interaction inhibits the influx of calcium ions into the neurons, thereby influencing various cellular processes, including enzyme activation and changes in gene expression .
Temporal Effects in Laboratory Settings
The effects of 1-Naphthylacetylspermine can change over time in laboratory settings
Dosage Effects in Animal Models
The effects of 1-Naphthylacetylspermine can vary with different dosages in animal models
Metabolic Pathways
Given its role as an AMPA receptor antagonist, it is likely to be involved in pathways related to neuronal signaling and calcium ion homeostasis .
Transport and Distribution
Given its role as an AMPA receptor antagonist, it is likely to be localized in areas where these receptors are present .
Subcellular Localization
Given its role as an AMPA receptor antagonist, it is likely to be localized in areas where these receptors are present .
Preparation Methods
Synthetic Routes and Reaction Conditions
1-Naphthylacetyl spermine is synthesized through a series of chemical reactions involving the acetylation of spermine with 1-naphthylacetyl chloride. The reaction typically requires anhydrous conditions and a suitable solvent such as dichloromethane. The reaction is carried out at low temperatures to ensure the stability of the intermediate products .
Industrial Production Methods
While specific industrial production methods for 1-naphthylacetyl spermine are not widely documented, the synthesis generally follows the same principles as laboratory preparation. Large-scale production would involve optimization of reaction conditions to maximize yield and purity, as well as the implementation of rigorous quality control measures to ensure consistency .
Chemical Reactions Analysis
Types of Reactions
1-Naphthylacetyl spermine primarily undergoes substitution reactions due to the presence of reactive amine groups. It can also participate in oxidation and reduction reactions under specific conditions .
Common Reagents and Conditions
Substitution Reactions: Typically involve reagents such as acyl chlorides or anhydrides in the presence of a base like triethylamine.
Oxidation Reactions: Can be carried out using oxidizing agents such as hydrogen peroxide or potassium permanganate.
Reduction Reactions: Often involve reducing agents like sodium borohydride or lithium aluminum hydride.
Major Products
The major products formed from these reactions depend on the specific reagents and conditions used. For example, acetylation of spermine with 1-naphthylacetyl chloride yields 1-naphthylacetyl spermine .
Scientific Research Applications
1-Naphthylacetyl spermine is widely used in scientific research, particularly in the fields of neuroscience and pharmacology. Its primary application is in the study of calcium-permeable AMPA receptors, which play a crucial role in synaptic plasticity and various neurological disorders . The compound is also used to investigate the mechanisms of excitotoxicity and neuroprotection, making it valuable in the development of therapeutic strategies for conditions such as stroke and neurodegenerative diseases .
Comparison with Similar Compounds
1-Naphthylacetyl spermine is unique in its selective inhibition of calcium-permeable AMPA receptors. Similar compounds include:
Spermine: A naturally occurring polyamine that also blocks calcium-permeable AMPA receptors but with less specificity.
Philanthotoxin-433: Another synthetic analogue of Joro spider toxin with a different distribution of amine groups, offering distinct blocking properties.
Philanthotoxin-74: Lacks one amine group compared to 1-naphthylacetyl spermine, resulting in different pharmacological effects.
These compounds highlight the specificity and potency of 1-naphthylacetyl spermine in targeting calcium-permeable AMPA receptors, making it a valuable tool in neuroscience research .
Properties
IUPAC Name |
N-[3-[4-(3-aminopropylamino)butylamino]propyl]-2-naphthalen-1-ylacetamide | |
---|---|---|
Source | PubChem | |
URL | https://pubchem.ncbi.nlm.nih.gov | |
Description | Data deposited in or computed by PubChem | |
InChI |
InChI=1S/C22H34N4O/c23-12-6-15-24-13-3-4-14-25-16-7-17-26-22(27)18-20-10-5-9-19-8-1-2-11-21(19)20/h1-2,5,8-11,24-25H,3-4,6-7,12-18,23H2,(H,26,27) | |
Source | PubChem | |
URL | https://pubchem.ncbi.nlm.nih.gov | |
Description | Data deposited in or computed by PubChem | |
InChI Key |
ZUINPPQIQARTKX-UHFFFAOYSA-N | |
Source | PubChem | |
URL | https://pubchem.ncbi.nlm.nih.gov | |
Description | Data deposited in or computed by PubChem | |
Canonical SMILES |
C1=CC=C2C(=C1)C=CC=C2CC(=O)NCCCNCCCCNCCCN | |
Source | PubChem | |
URL | https://pubchem.ncbi.nlm.nih.gov | |
Description | Data deposited in or computed by PubChem | |
Molecular Formula |
C22H34N4O | |
Source | PubChem | |
URL | https://pubchem.ncbi.nlm.nih.gov | |
Description | Data deposited in or computed by PubChem | |
DSSTOX Substance ID |
DTXSID80153568 | |
Record name | 1-Naphthylacetylspermine | |
Source | EPA DSSTox | |
URL | https://comptox.epa.gov/dashboard/DTXSID80153568 | |
Description | DSSTox provides a high quality public chemistry resource for supporting improved predictive toxicology. | |
Molecular Weight |
370.5 g/mol | |
Source | PubChem | |
URL | https://pubchem.ncbi.nlm.nih.gov | |
Description | Data deposited in or computed by PubChem | |
CAS No. |
122306-11-0 | |
Record name | N-[3-[[4-[(3-Aminopropyl)amino]butyl]amino]propyl]-1-naphthaleneacetamide | |
Source | CAS Common Chemistry | |
URL | https://commonchemistry.cas.org/detail?cas_rn=122306-11-0 | |
Description | CAS Common Chemistry is an open community resource for accessing chemical information. Nearly 500,000 chemical substances from CAS REGISTRY cover areas of community interest, including common and frequently regulated chemicals, and those relevant to high school and undergraduate chemistry classes. This chemical information, curated by our expert scientists, is provided in alignment with our mission as a division of the American Chemical Society. | |
Explanation | The data from CAS Common Chemistry is provided under a CC-BY-NC 4.0 license, unless otherwise stated. | |
Record name | 1-Naphthylacetylspermine | |
Source | ChemIDplus | |
URL | https://pubchem.ncbi.nlm.nih.gov/substance/?source=chemidplus&sourceid=0122306110 | |
Description | ChemIDplus is a free, web search system that provides access to the structure and nomenclature authority files used for the identification of chemical substances cited in National Library of Medicine (NLM) databases, including the TOXNET system. | |
Record name | 1-Naphthylacetylspermine | |
Source | EPA DSSTox | |
URL | https://comptox.epa.gov/dashboard/DTXSID80153568 | |
Description | DSSTox provides a high quality public chemistry resource for supporting improved predictive toxicology. | |
Retrosynthesis Analysis
AI-Powered Synthesis Planning: Our tool employs the Template_relevance Pistachio, Template_relevance Bkms_metabolic, Template_relevance Pistachio_ringbreaker, Template_relevance Reaxys, Template_relevance Reaxys_biocatalysis model, leveraging a vast database of chemical reactions to predict feasible synthetic routes.
One-Step Synthesis Focus: Specifically designed for one-step synthesis, it provides concise and direct routes for your target compounds, streamlining the synthesis process.
Accurate Predictions: Utilizing the extensive PISTACHIO, BKMS_METABOLIC, PISTACHIO_RINGBREAKER, REAXYS, REAXYS_BIOCATALYSIS database, our tool offers high-accuracy predictions, reflecting the latest in chemical research and data.
Strategy Settings
Precursor scoring | Relevance Heuristic |
---|---|
Min. plausibility | 0.01 |
Model | Template_relevance |
Template Set | Pistachio/Bkms_metabolic/Pistachio_ringbreaker/Reaxys/Reaxys_biocatalysis |
Top-N result to add to graph | 6 |
Feasible Synthetic Routes
Disclaimer and Information on In-Vitro Research Products
Please be aware that all articles and product information presented on BenchChem are intended solely for informational purposes. The products available for purchase on BenchChem are specifically designed for in-vitro studies, which are conducted outside of living organisms. In-vitro studies, derived from the Latin term "in glass," involve experiments performed in controlled laboratory settings using cells or tissues. It is important to note that these products are not categorized as medicines or drugs, and they have not received approval from the FDA for the prevention, treatment, or cure of any medical condition, ailment, or disease. We must emphasize that any form of bodily introduction of these products into humans or animals is strictly prohibited by law. It is essential to adhere to these guidelines to ensure compliance with legal and ethical standards in research and experimentation.