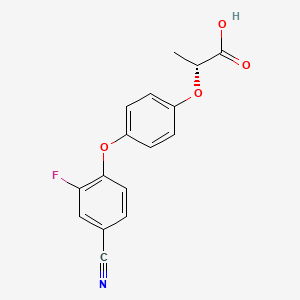
Cyhalofop
Overview
Description
Cyhalofop-butyl [(R)-2-[4-(4-cyano-2-fluorophenoxy)phenoxy]propanoic acid butyl ester] is a post-emergence aryloxyphenoxypropionate (APP) herbicide widely used to control grass weeds in rice paddies. It inhibits acetyl-CoA carboxylase (ACCase), a key enzyme in fatty acid biosynthesis . Upon absorption, this compound-butyl is rapidly hydrolyzed to its active form, this compound acid, which disrupts lipid synthesis in susceptible plants . Resistance to this compound in weeds like Echinochloa crus-galli (barnyardgrass) is attributed to both target-site mutations (e.g., ACCase gene overexpression) and non-target-site mechanisms, including enhanced metabolic detoxification via cytochrome P450 monooxygenases (P450s) and glutathione S-transferases (GSTs) .
Preparation Methods
The synthesis of cyhalofop-butyl involves several steps:
Dissolution: R-2-(4-hydroxyphenoxy) propionic acid is dissolved in an organic solvent along with a catalyst to form a mixed solution.
Addition of Acid-Binding Agent: An acid-binding agent is slowly added to the solution until no gas is generated, forming a new mixed solution.
Reaction with Bromobutane: Bromobutane is added to the solution, and the reaction is carried out at 85-95°C to produce an intermediate, R-2-(4-hydroxyphenoxy) butyl propionate.
Final Reaction: 3,4-difluorobenzonitrile is added to the intermediate, maintaining the temperature to generate the final product, this compound-butyl.
Chemical Reactions Analysis
Etherification Reaction
Reaction conditions and catalysts significantly impact yield and optical purity:
Catalyst | Solvent | Temp (°C) | Yield (%) | Enantiomeric Excess (e.e. %) | Source |
---|---|---|---|---|---|
None | DMF | 80 | 72 | - | |
N(Bu)₄HSO₄ | DMF | 80 | 94 | 98 | |
N(Bu)₄Br | DMF | 80 | 92 | 92 | |
TEBA | DMF | 80 | 92 | 95 |
Key Findings :
-
Phase-transfer catalysts (e.g., tetrabutylammonium hydrogen sulfate, N(Bu)₄HSO₄) enhance both yield (94%) and enantioselectivity (98% e.e.) by facilitating ion-pair transfer in biphasic systems .
-
Solvent dependency : DMF outperforms acetonitrile, DMAc, and nonpolar solvents (e.g., toluene) due to superior solubility of reactants and base (K₂CO₃) .
-
Temperature sensitivity : Reactions above 100°C reduce optical purity due to racemization under alkaline conditions .
Esterification Reaction
The intermediate acid undergoes esterification with butyl bromide:
-
Stoichiometry : A 3:1 molar excess of butyl bromide is required for >95% yield; lower ratios (2:1) yield 82% .
-
Product isolation : Excess butyl bromide is recovered via distillation .
Resorcinol-Based Pathway
-
Step 1 : Resorcinol reacts with 3,4-difluorobenzonitrile to form 3-fluoro-4-(4-hydroxyphenoxy)benzonitrile.
-
Step 2 : Alkylation with (S)-tosyl lactate yields this compound-butyl.
Limitations : -
High cost due to 3,4-difluorobenzonitrile consumption and byproduct formation .
Racemization Mitigation Strategies
-
Low-temperature catalysis : Maintaining reactions at 80°C prevents racemization while achieving >90% conversion .
-
Chiral retention : Phase-transfer catalysts stabilize the transition state, preserving (R)-configuration .
Reaction Byproducts and Challenges
-
Byproducts : Biphenol derivatives form due to resorcinol’s dual phenolic hydroxyl groups, increasing 3,4-difluorobenzonitrile consumption .
-
Cost drivers : 3,4-Difluorobenzonitrile accounts for ~70% of raw material costs, necessitating high-yield protocols .
Environmental Degradation Pathways
While not a synthesis reaction, environmental breakdown includes:
Industrial-Scale Optimization
-
Catalyst recycling : Tetrabutylammonium salts are recovered via aqueous extraction .
-
Solvent selection : DMF is preferred for its balance of reactivity and cost .
This synthesis framework highlights the interplay between catalytic innovation, solvent engineering, and stoichiometric precision to achieve high-purity this compound-butyl. Experimental data from controlled studies provide actionable insights for industrial applications, emphasizing phase-transfer catalysis as the gold standard for enantioselective synthesis.
Scientific Research Applications
Cyhalofop is a chemical compound primarily utilized as a selective herbicide in agriculture, particularly for rice cultivation3 . Its main application is to control grass weeds, ensuring the healthy growth of rice crops3.
Chemical Description
This compound-butyl is an aryloxyphenoxy propionate herbicide . The compound's chemical name is butyl (R) -2-[4-(4-cyano-2-fluorophenoxy) phenoxy] propionate .
Mode of Action
This compound-butyl works by inhibiting the activity of serine threonine protein phosphatase (STPP), an enzyme crucial for the growth and development of grass weeds3. By disrupting this enzyme, this compound-butyl interferes with important cellular processes, ultimately leading to the death of the targeted grass weeds3.
Formulation and Application
This compound-butyl is typically formulated as an emulsifiable concentrate (EC) or a suspension concentrate (SC)3. These formulations allow for easy mixing and application, ensuring effective weed control in various agricultural settings3. The recommended dosage of this compound-butyl varies depending on the target weed species, crop, and local regulations3. For foliar applications in rice, the dose ranges from 100 to 500 grams of active ingredient per hectare3.
Agricultural Applications
This compound-butyl is primarily used to control grass weeds in crops such as rice3. It effectively targets grass weeds like barnyard grass, wild oats, ryegrass, and Johnson grass3. Additionally, it controls Leptocloa species and other grasses in rice fields3. this compound-butyl provides selective control, allowing for the suppression of grass weeds while preserving the growth of rice plants3.
Benefits
This compound-butyl exhibits high activity and excellent selectivity for killing weeds, with the advantage of low toxicity and minimal residue . Due to its low application rates, this compound-butyl is considered a highly efficient, environmentally benign, and safe herbicide, as it is mildly hydrolyzed under neutral or weak acid environments .
Resistance Mechanisms
Resistance to this compound-butyl has been observed in some grass-weed populations . Studies have identified diverse mechanisms associated with this compound-butyl resistance, including alterations in ACCase mutations . For example, a Trp 2027-Cys substitution might explain this compound-butyl resistance in certain L. chinensis populations .
Environmental Considerations
While this compound-butyl is generally considered safe, research indicates potential environmental impacts. For instance, this compound-butyl exposure can induce oxidative stress and muscle damage in certain aquatic species . One study showed that exposure to this compound-butyl can significantly alter metabolism-related pathways in P. clarkii, affecting drug metabolism, glutathione metabolism, fatty acid biosynthesis, and fatty acid degradation .
Regulatory Information
Mechanism of Action
Cyhalofop exerts its herbicidal effects by inhibiting the enzyme acetyl coenzyme A carboxylase (ACCase). This enzyme is pivotal in the biosynthesis of fatty acids, which are essential for cell membrane formation and energy storage in plants. By inhibiting ACCase, this compound disrupts fatty acid synthesis, leading to the death of the targeted grass weeds .
Comparison with Similar Compounds
ACCase-Inhibiting Herbicides
Metamifop
- Chemical Profile : A newer APP herbicide with structural similarities to cyhalofop.
- Efficacy : Metamifop exhibits stronger binding affinity to LcCYP76C1–5 (a detoxification enzyme in Leptochloa chinensis), with a binding energy of −7.39 kcal/mol compared to this compound acid’s −6.18 kcal/mol . This suggests metamifop may face faster metabolic resistance in weeds.
- Resistance Mechanisms : Both herbicides induce P450-mediated detoxification, but this compound resistance in barnyardgrass is linked to ACCase1/ACCase3 overexpression, whereas metamifop resistance involves CYP76C1 gene amplification .
Fenoxaprop-P-ethyl
- Efficacy: Fenoxaprop-P-ethyl shows broader-spectrum activity against grasses but lower stability under flooded conditions compared to this compound .
- Environmental Persistence: this compound’s anionic form (pKa 3.9) reduces sorption to biochar, leading to higher mobility in soil, whereas fenoxaprop’s ester form exhibits moderate adsorption .
Non-ACCase Herbicides with Overlapping Use Cases
Propanil
- Mode of Action : A photosystem II inhibitor.
- Resistance Trends: Propanil resistance in Echinochloa is widespread (53% of tested populations) compared to this compound (12%) due to prolonged use .
- Environmental Stability : Propanil degrades rapidly via hydrolysis, whereas this compound’s persistence is pH-dependent, with reduced efficacy in alkaline soils .
Clomazone
- Mode of Action: A carotenoid biosynthesis inhibitor.
- Sorption Behavior: Clomazone has 3–10× higher sorption to biochar (RE = 65–70%) than this compound (RE = 6–35%) due to its non-ionic structure and hydrophobic interactions .
Data Tables
Table 1: Efficacy and Resistance Profiles of Herbicides
Resistance prevalence in *Echinochloa spp.
Table 2: Physicochemical and Environmental Properties
Herbicide | pKa | Sorption (Koc) | Dominant Form in Soil | Biochar Sorption (RE%) |
---|---|---|---|---|
This compound acid | 3.9 | 186 | Anionic | 6–35 |
Clomazone | Non-ionic | 300 | Non-ionic | 65–70 |
Propanil | 1.8 | 50–100 | Neutral | <10 |
Key Research Findings
Metabolic Resistance : this compound-resistant barnyardgrass reduces this compound acid content by 63–67% via P450/GST-mediated detoxification, a mechanism less common in propanil-resistant weeds .
Target-Site vs. Non-Target Resistance: ACCase gene amplification is rare in this compound resistance, contrasting with metamifop, where CYP76C1 amplification is prevalent .
Environmental Sensitivity : this compound efficacy drops under high pH or flooding, whereas quinclorac remains stable but faces higher resistance risks .
Biological Activity
Cyhalofop-butyl is a selective herbicide primarily used for controlling grass weeds in rice paddies. Its biological activity encompasses a range of effects on various organisms, including plants and aquatic life. This article consolidates findings from multiple studies to provide an authoritative overview of the biological activity of this compound-butyl, focusing on its mechanisms of action, toxicity, resistance development, and ecological implications.
This compound-butyl functions as an acetyl-CoA carboxylase (ACCase) inhibitor, disrupting fatty acid synthesis in susceptible plant species. This inhibition leads to the cessation of growth and eventual death of target weeds. The herbicide's efficacy is particularly notable against Leptochloa chinensis (barnyardgrass), a common weed in rice cultivation.
Mechanism | Description |
---|---|
ACCase Inhibition | Blocks fatty acid synthesis, leading to plant growth inhibition |
Target Species | Primarily effective against grass weeds like L. chinensis |
Resistance Development | Mutations in ACCase genes can confer resistance in certain populations |
Toxicological Effects
Recent studies have highlighted the hepatotoxic and immunotoxic effects of this compound-butyl, particularly in aquatic organisms such as zebrafish. Exposure to this herbicide has been shown to induce significant liver damage and affect immune responses.
Case Study: Zebrafish Embryo Exposure
In a study examining the effects of this compound-butyl on zebrafish embryos, researchers found:
- Hepatotoxicity : Significant liver damage was observed at concentrations as low as 0.166 mg/L, with increased activity of liver enzymes indicating cellular stress.
- Immunotoxicity : The herbicide exposure led to alterations in immune cell populations, suggesting potential long-term impacts on fish health .
Table 2: Toxicological Effects Observed in Zebrafish
Endpoint | Concentration (mg/L) | Effect Observed |
---|---|---|
Liver Enzyme Activity | 0.166 | Increased activity of caspases |
Immune Cell Alteration | 0.333 | Significant changes in immune profiles |
Resistance Mechanisms
The development of resistance to this compound-butyl has been documented in various weed populations. Mechanisms include target site resistance (TSR) through mutations in the ACCase gene and metabolic resistance involving cytochrome P450 enzymes and glutathione S-transferases (GSTs).
Findings from Recent Research
- Mutational Analysis : Studies identified specific mutations (e.g., Trp 2027-Cys) associated with resistance in L. chinensis populations .
- Metabolic Resistance : Upregulation of P450 and GST genes was linked to enhanced detoxification capabilities in resistant biotypes .
Table 3: Resistance Mechanisms Identified
Mechanism | Description |
---|---|
Target Site Resistance | Mutations in ACCase gene leading to reduced herbicide binding |
Metabolic Resistance | Enhanced detoxification via P450s and GSTs |
Ecological Implications
The widespread use of this compound-butyl raises concerns about its ecological impact, particularly on non-target species. The toxicity observed in aquatic organisms suggests potential risks for ecosystems surrounding agricultural areas.
Summary of Ecological Risks
- Aquatic Toxicity : Significant adverse effects on fish and other aquatic organisms.
- Resistance Development : Potential for increased herbicide use leading to further ecological imbalances.
Q & A
Basic Research Questions
Q. What are the primary biochemical pathways targeted by Cyhalofop in grass weeds, and how can researchers design experiments to quantify enzyme inhibition?
this compound inhibits acetyl-CoA carboxylase (ACCase), a critical enzyme in lipid biosynthesis. To study this, researchers can use in vitro enzyme assays with purified ACCase from susceptible and resistant weed species. Include controls with ACCase inhibitors (e.g., diclofop-methyl) to validate specificity. Measure enzyme activity via spectrophotometric detection of NADH consumption or malonyl-CoA production .
Q. What standardized protocols exist for evaluating this compound’s efficacy in controlled agricultural trials?
Follow dose-response experiments in greenhouse or field settings using randomized block designs. Apply this compound at varying concentrations (e.g., 50–400 g ai/ha) to juvenile grass weeds (e.g., Echinochloa crus-galli). Assess mortality rates, biomass reduction, and chlorophyll content at 7, 14, and 21 days post-application. Use ANOVA to compare treatment effects and calculate ED₅₀ values .
Q. How can researchers assess this compound’s environmental persistence in soil and water systems?
Conduct soil half-life studies under controlled conditions (e.g., 25°C, 70% field capacity). Extract this compound and its metabolites (e.g., this compound-acid) using QuEChERS or solid-phase extraction, followed by quantification via HPLC-MS/MS. For aquatic systems, monitor degradation kinetics in water-sediment microcosms under aerobic/anaerobic conditions. Include abiotic controls to distinguish microbial vs. chemical degradation .
Advanced Research Questions
Q. What molecular mechanisms underlie this compound resistance in weeds, and how can CRISPR-Cas9 be used to validate target-site mutations?
Resistance often arises from ACCase gene mutations (e.g., Ile-1781-Leu). Amplify ACCase domains from resistant biotypes via PCR and sequence them to identify mutations. Use CRISPR-Cas9 to introduce suspected mutations into susceptible plant models (e.g., Arabidopsis). Compare herbicide sensitivity between wild-type and edited lines to confirm causal mutations .
Q. How can transcriptomic and metabolomic approaches elucidate non-target-site resistance (NTSR) to this compound?
Perform RNA-seq on resistant vs. susceptible weeds to identify upregulated detoxification genes (e.g., cytochrome P450s, GSTs). Validate findings with qRT-PCR. For metabolomics, use LC-HRMS to profile conjugates (e.g., glutathione-Cyhalofop adducts). Integrate datasets with bioinformatics tools like KEGG pathway analysis to map metabolic shifts .
Q. What synergistic effects occur when this compound is combined with auxin herbicides, and how can isobologram analysis optimize mixture ratios?
Test binary mixtures (e.g., this compound + 2,4-D) in dose-additivity experiments. Calculate the combined effect using Colby’s method or isobologram analysis. Fit data to a logistic model to determine synergistic/antagonistic indices. Validate field efficacy in plots with mixed weed populations (broadleaf + grass) .
Q. How do soil microbiota influence this compound degradation, and what metagenomic tools can identify key degrading organisms?
Incubate this compound-treated soil samples and extract DNA at intervals. Perform shotgun metagenomics or 16S rRNA sequencing to track microbial community shifts. Enrich consortia in minimal media with this compound as the sole carbon source. Identify degradative pathways via genome-resolved metagenomics and heterologous expression of putative hydrolase genes .
Q. Methodological Considerations
- Data Contradictions : When conflicting results arise (e.g., variable ED₅₀ values across studies), re-evaluate experimental conditions (e.g., plant growth stage, application timing) and validate assays with reference standards .
- Statistical Rigor : Use mixed-effects models to account for spatial variability in field trials. Report confidence intervals for degradation half-lives and resistance allele frequencies .
Properties
IUPAC Name |
(2R)-2-[4-(4-cyano-2-fluorophenoxy)phenoxy]propanoic acid | |
---|---|---|
Source | PubChem | |
URL | https://pubchem.ncbi.nlm.nih.gov | |
Description | Data deposited in or computed by PubChem | |
InChI |
InChI=1S/C16H12FNO4/c1-10(16(19)20)21-12-3-5-13(6-4-12)22-15-7-2-11(9-18)8-14(15)17/h2-8,10H,1H3,(H,19,20)/t10-/m1/s1 | |
Source | PubChem | |
URL | https://pubchem.ncbi.nlm.nih.gov | |
Description | Data deposited in or computed by PubChem | |
InChI Key |
ROBSGBGTWRRYSK-SNVBAGLBSA-N | |
Source | PubChem | |
URL | https://pubchem.ncbi.nlm.nih.gov | |
Description | Data deposited in or computed by PubChem | |
Canonical SMILES |
CC(C(=O)O)OC1=CC=C(C=C1)OC2=C(C=C(C=C2)C#N)F | |
Source | PubChem | |
URL | https://pubchem.ncbi.nlm.nih.gov | |
Description | Data deposited in or computed by PubChem | |
Isomeric SMILES |
C[C@H](C(=O)O)OC1=CC=C(C=C1)OC2=C(C=C(C=C2)C#N)F | |
Source | PubChem | |
URL | https://pubchem.ncbi.nlm.nih.gov | |
Description | Data deposited in or computed by PubChem | |
Molecular Formula |
C16H12FNO4 | |
Source | PubChem | |
URL | https://pubchem.ncbi.nlm.nih.gov | |
Description | Data deposited in or computed by PubChem | |
DSSTOX Substance ID |
DTXSID701022032 | |
Record name | Cyhalofop | |
Source | EPA DSSTox | |
URL | https://comptox.epa.gov/dashboard/DTXSID701022032 | |
Description | DSSTox provides a high quality public chemistry resource for supporting improved predictive toxicology. | |
Molecular Weight |
301.27 g/mol | |
Source | PubChem | |
URL | https://pubchem.ncbi.nlm.nih.gov | |
Description | Data deposited in or computed by PubChem | |
CAS No. |
122008-78-0 | |
Record name | Cyhalofop [ISO] | |
Source | ChemIDplus | |
URL | https://pubchem.ncbi.nlm.nih.gov/substance/?source=chemidplus&sourceid=0122008780 | |
Description | ChemIDplus is a free, web search system that provides access to the structure and nomenclature authority files used for the identification of chemical substances cited in National Library of Medicine (NLM) databases, including the TOXNET system. | |
Record name | Cyhalofop | |
Source | EPA DSSTox | |
URL | https://comptox.epa.gov/dashboard/DTXSID701022032 | |
Description | DSSTox provides a high quality public chemistry resource for supporting improved predictive toxicology. | |
Record name | Cyhalofop | |
Source | European Chemicals Agency (ECHA) | |
URL | https://echa.europa.eu/information-on-chemicals | |
Description | The European Chemicals Agency (ECHA) is an agency of the European Union which is the driving force among regulatory authorities in implementing the EU's groundbreaking chemicals legislation for the benefit of human health and the environment as well as for innovation and competitiveness. | |
Explanation | Use of the information, documents and data from the ECHA website is subject to the terms and conditions of this Legal Notice, and subject to other binding limitations provided for under applicable law, the information, documents and data made available on the ECHA website may be reproduced, distributed and/or used, totally or in part, for non-commercial purposes provided that ECHA is acknowledged as the source: "Source: European Chemicals Agency, http://echa.europa.eu/". Such acknowledgement must be included in each copy of the material. ECHA permits and encourages organisations and individuals to create links to the ECHA website under the following cumulative conditions: Links can only be made to webpages that provide a link to the Legal Notice page. | |
Record name | CYHALOFOP | |
Source | FDA Global Substance Registration System (GSRS) | |
URL | https://gsrs.ncats.nih.gov/ginas/app/beta/substances/58150F267D | |
Description | The FDA Global Substance Registration System (GSRS) enables the efficient and accurate exchange of information on what substances are in regulated products. Instead of relying on names, which vary across regulatory domains, countries, and regions, the GSRS knowledge base makes it possible for substances to be defined by standardized, scientific descriptions. | |
Explanation | Unless otherwise noted, the contents of the FDA website (www.fda.gov), both text and graphics, are not copyrighted. They are in the public domain and may be republished, reprinted and otherwise used freely by anyone without the need to obtain permission from FDA. Credit to the U.S. Food and Drug Administration as the source is appreciated but not required. | |
Retrosynthesis Analysis
AI-Powered Synthesis Planning: Our tool employs the Template_relevance Pistachio, Template_relevance Bkms_metabolic, Template_relevance Pistachio_ringbreaker, Template_relevance Reaxys, Template_relevance Reaxys_biocatalysis model, leveraging a vast database of chemical reactions to predict feasible synthetic routes.
One-Step Synthesis Focus: Specifically designed for one-step synthesis, it provides concise and direct routes for your target compounds, streamlining the synthesis process.
Accurate Predictions: Utilizing the extensive PISTACHIO, BKMS_METABOLIC, PISTACHIO_RINGBREAKER, REAXYS, REAXYS_BIOCATALYSIS database, our tool offers high-accuracy predictions, reflecting the latest in chemical research and data.
Strategy Settings
Precursor scoring | Relevance Heuristic |
---|---|
Min. plausibility | 0.01 |
Model | Template_relevance |
Template Set | Pistachio/Bkms_metabolic/Pistachio_ringbreaker/Reaxys/Reaxys_biocatalysis |
Top-N result to add to graph | 6 |
Feasible Synthetic Routes
Disclaimer and Information on In-Vitro Research Products
Please be aware that all articles and product information presented on BenchChem are intended solely for informational purposes. The products available for purchase on BenchChem are specifically designed for in-vitro studies, which are conducted outside of living organisms. In-vitro studies, derived from the Latin term "in glass," involve experiments performed in controlled laboratory settings using cells or tissues. It is important to note that these products are not categorized as medicines or drugs, and they have not received approval from the FDA for the prevention, treatment, or cure of any medical condition, ailment, or disease. We must emphasize that any form of bodily introduction of these products into humans or animals is strictly prohibited by law. It is essential to adhere to these guidelines to ensure compliance with legal and ethical standards in research and experimentation.