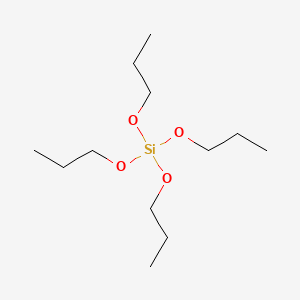
Tetrapropyl orthosilicate
Overview
Description
Mechanism of Action
Target of Action
Tetrapropoxysilane primarily targets the formation of chemical bonds between various materials . It acts as a vital crosslinking agent in the formulation of coatings, sealants, adhesives, and other chemical products .
Mode of Action
Tetrapropoxysilane interacts with its targets by promoting covalent bonding between the coating material and the substrate . Its unique chemical structure allows it to form strong chemical bonds, resulting in improved bond strength .
Biochemical Pathways
The Lewis acid-catalysed Piers-Rubinsztajn (PR) reaction of hydride-terminated siloxanes with various tetrafunctional alkoxysilanes is a key biochemical pathway influenced by Tetrapropoxysilane . This reaction process can be tailored to reliably produce homogeneous, polysiloxane network materials .
Pharmacokinetics
It has a molecular weight of 264.43, a density of 0.916 g/mL at 25 °C, and a boiling point of 94 °C/5 mmHg . These properties may influence its bioavailability and pharmacokinetics.
Result of Action
The result of Tetrapropoxysilane’s action is the formation of a stronger and more durable bond, preventing the coating from delaminating or peeling off under external stresses such as temperature variations, physical impacts, or chemical exposure . It significantly improves the adhesion between the coating and the substrate, resulting in enhanced durability and longevity .
Action Environment
Environmental factors can influence the action, efficacy, and stability of Tetrapropoxysilane. For instance, it has been shown to provide excellent hydrolysis resistance, ensuring that the coated surface remains intact even in high humidity environments . Furthermore, the rate of the curing reaction can be influenced by electronics and sterics .
Preparation Methods
Tetrapropyl orthosilicate is typically synthesized through the reaction of silicon tetrachloride with propanol in the presence of a catalyst such as hydrochloric acid or aluminum chloride . The reaction produces this compound and hydrogen chloride gas, which is then neutralized with a base like sodium hydroxide or potassium hydroxide .
In industrial settings, the sol-gel process is commonly used to produce this compound. This method involves the hydrolysis and condensation of silicon alkoxides in the presence of water and a catalyst . The sol-gel process allows for the control of particle size and pore structure, making it suitable for the production of monodisperse mesoporous silica particles .
Chemical Reactions Analysis
Tetrapropyl orthosilicate undergoes various chemical reactions, including hydrolysis and condensation. In the presence of water, it hydrolyzes to form silicon dioxide (silica) and propanol . This reaction is typically catalyzed by acids or bases, with hydrochloric acid and ammonia being common choices .
The compound can also participate in sol-gel reactions, where it forms a three-dimensional network of silica through hydrolysis and polycondensation . These reactions are often carried out under controlled conditions to achieve the desired properties of the final product .
Scientific Research Applications
Tetrapropyl orthosilicate has a wide range of applications in scientific research and industry. In chemistry, it is used as a precursor for the synthesis of silica-based materials, including mesoporous silica particles and aerogels . These materials are valuable for their high surface area, porosity, and thermal stability, making them suitable for applications in catalysis, adsorption, and separation processes .
In biology and medicine, this compound is used in the development of biocompatible materials for drug delivery and tissue engineering .
In the industrial sector, this compound is employed as a cross-linking agent for silicon rubber and as a modifying agent for organic and inorganic resins . It is also used in the production of corrosion-resistant coatings and as a binder in refractory materials .
Comparison with Similar Compounds
Tetrapropyl orthosilicate is part of the tetraalkyl orthosilicate family, which includes compounds like tetramethyl orthosilicate, tetraethyl orthosilicate, and tetrabutyl orthosilicate . These compounds differ in the length of their alkyl chains, which affects their hydrolysis rates and the properties of the resulting silica .
Tetramethyl orthosilicate (TMOS): Hydrolyzes rapidly to form silica and methanol.
Tetraethyl orthosilicate (TEOS): Hydrolyzes to form silica and ethanol.
Tetrabutyl orthosilicate (TBOS): Hydrolyzes more slowly than TMOS and TEOS, producing silica and butanol.
This compound’s unique properties, such as its slower hydrolysis rate and the ability to form well-defined silica structures, make it particularly useful in applications where controlled hydrolysis and condensation are essential .
Properties
IUPAC Name |
tetrapropyl silicate | |
---|---|---|
Source | PubChem | |
URL | https://pubchem.ncbi.nlm.nih.gov | |
Description | Data deposited in or computed by PubChem | |
InChI |
InChI=1S/C12H28O4Si/c1-5-9-13-17(14-10-6-2,15-11-7-3)16-12-8-4/h5-12H2,1-4H3 | |
Source | PubChem | |
URL | https://pubchem.ncbi.nlm.nih.gov | |
Description | Data deposited in or computed by PubChem | |
InChI Key |
ZQZCOBSUOFHDEE-UHFFFAOYSA-N | |
Source | PubChem | |
URL | https://pubchem.ncbi.nlm.nih.gov | |
Description | Data deposited in or computed by PubChem | |
Canonical SMILES |
CCCO[Si](OCCC)(OCCC)OCCC | |
Source | PubChem | |
URL | https://pubchem.ncbi.nlm.nih.gov | |
Description | Data deposited in or computed by PubChem | |
Molecular Formula |
C12H28O4Si | |
Source | PubChem | |
URL | https://pubchem.ncbi.nlm.nih.gov | |
Description | Data deposited in or computed by PubChem | |
DSSTOX Substance ID |
DTXSID9042382 | |
Record name | Tetrapropyl orthosilicate | |
Source | EPA DSSTox | |
URL | https://comptox.epa.gov/dashboard/DTXSID9042382 | |
Description | DSSTox provides a high quality public chemistry resource for supporting improved predictive toxicology. | |
Molecular Weight |
264.43 g/mol | |
Source | PubChem | |
URL | https://pubchem.ncbi.nlm.nih.gov | |
Description | Data deposited in or computed by PubChem | |
Physical Description |
Liquid, Colorless liquid; [Alfa Aesar MSDS] | |
Record name | Silicic acid (H4SiO4), tetrapropyl ester | |
Source | EPA Chemicals under the TSCA | |
URL | https://www.epa.gov/chemicals-under-tsca | |
Description | EPA Chemicals under the Toxic Substances Control Act (TSCA) collection contains information on chemicals and their regulations under TSCA, including non-confidential content from the TSCA Chemical Substance Inventory and Chemical Data Reporting. | |
Record name | Tetrapropoxysilane | |
Source | Haz-Map, Information on Hazardous Chemicals and Occupational Diseases | |
URL | https://haz-map.com/Agents/17957 | |
Description | Haz-Map® is an occupational health database designed for health and safety professionals and for consumers seeking information about the adverse effects of workplace exposures to chemical and biological agents. | |
Explanation | Copyright (c) 2022 Haz-Map(R). All rights reserved. Unless otherwise indicated, all materials from Haz-Map are copyrighted by Haz-Map(R). No part of these materials, either text or image may be used for any purpose other than for personal use. Therefore, reproduction, modification, storage in a retrieval system or retransmission, in any form or by any means, electronic, mechanical or otherwise, for reasons other than personal use, is strictly prohibited without prior written permission. | |
CAS No. |
682-01-9 | |
Record name | Tetrapropoxysilane | |
Source | CAS Common Chemistry | |
URL | https://commonchemistry.cas.org/detail?cas_rn=682-01-9 | |
Description | CAS Common Chemistry is an open community resource for accessing chemical information. Nearly 500,000 chemical substances from CAS REGISTRY cover areas of community interest, including common and frequently regulated chemicals, and those relevant to high school and undergraduate chemistry classes. This chemical information, curated by our expert scientists, is provided in alignment with our mission as a division of the American Chemical Society. | |
Explanation | The data from CAS Common Chemistry is provided under a CC-BY-NC 4.0 license, unless otherwise stated. | |
Record name | Tetrapropyl orthosilicate | |
Source | ChemIDplus | |
URL | https://pubchem.ncbi.nlm.nih.gov/substance/?source=chemidplus&sourceid=0000682019 | |
Description | ChemIDplus is a free, web search system that provides access to the structure and nomenclature authority files used for the identification of chemical substances cited in National Library of Medicine (NLM) databases, including the TOXNET system. | |
Record name | Silicic acid (H4SiO4), tetrapropyl ester | |
Source | EPA Chemicals under the TSCA | |
URL | https://www.epa.gov/chemicals-under-tsca | |
Description | EPA Chemicals under the Toxic Substances Control Act (TSCA) collection contains information on chemicals and their regulations under TSCA, including non-confidential content from the TSCA Chemical Substance Inventory and Chemical Data Reporting. | |
Record name | Tetrapropyl orthosilicate | |
Source | EPA DSSTox | |
URL | https://comptox.epa.gov/dashboard/DTXSID9042382 | |
Description | DSSTox provides a high quality public chemistry resource for supporting improved predictive toxicology. | |
Record name | Tetrapropyl orthosilicate | |
Source | European Chemicals Agency (ECHA) | |
URL | https://echa.europa.eu/substance-information/-/substanceinfo/100.010.600 | |
Description | The European Chemicals Agency (ECHA) is an agency of the European Union which is the driving force among regulatory authorities in implementing the EU's groundbreaking chemicals legislation for the benefit of human health and the environment as well as for innovation and competitiveness. | |
Explanation | Use of the information, documents and data from the ECHA website is subject to the terms and conditions of this Legal Notice, and subject to other binding limitations provided for under applicable law, the information, documents and data made available on the ECHA website may be reproduced, distributed and/or used, totally or in part, for non-commercial purposes provided that ECHA is acknowledged as the source: "Source: European Chemicals Agency, http://echa.europa.eu/". Such acknowledgement must be included in each copy of the material. ECHA permits and encourages organisations and individuals to create links to the ECHA website under the following cumulative conditions: Links can only be made to webpages that provide a link to the Legal Notice page. | |
Record name | TETRAPROPYL ORTHOSILICATE | |
Source | FDA Global Substance Registration System (GSRS) | |
URL | https://gsrs.ncats.nih.gov/ginas/app/beta/substances/4PE821G3GH | |
Description | The FDA Global Substance Registration System (GSRS) enables the efficient and accurate exchange of information on what substances are in regulated products. Instead of relying on names, which vary across regulatory domains, countries, and regions, the GSRS knowledge base makes it possible for substances to be defined by standardized, scientific descriptions. | |
Explanation | Unless otherwise noted, the contents of the FDA website (www.fda.gov), both text and graphics, are not copyrighted. They are in the public domain and may be republished, reprinted and otherwise used freely by anyone without the need to obtain permission from FDA. Credit to the U.S. Food and Drug Administration as the source is appreciated but not required. | |
Retrosynthesis Analysis
AI-Powered Synthesis Planning: Our tool employs the Template_relevance Pistachio, Template_relevance Bkms_metabolic, Template_relevance Pistachio_ringbreaker, Template_relevance Reaxys, Template_relevance Reaxys_biocatalysis model, leveraging a vast database of chemical reactions to predict feasible synthetic routes.
One-Step Synthesis Focus: Specifically designed for one-step synthesis, it provides concise and direct routes for your target compounds, streamlining the synthesis process.
Accurate Predictions: Utilizing the extensive PISTACHIO, BKMS_METABOLIC, PISTACHIO_RINGBREAKER, REAXYS, REAXYS_BIOCATALYSIS database, our tool offers high-accuracy predictions, reflecting the latest in chemical research and data.
Strategy Settings
Precursor scoring | Relevance Heuristic |
---|---|
Min. plausibility | 0.01 |
Model | Template_relevance |
Template Set | Pistachio/Bkms_metabolic/Pistachio_ringbreaker/Reaxys/Reaxys_biocatalysis |
Top-N result to add to graph | 6 |
Feasible Synthetic Routes
Q1: How does Tetrapropoxysilane interact with its target, leading to desired downstream effects?
A1: Tetrapropoxysilane primarily interacts through hydrolysis and condensation reactions.
Q2: What is the molecular formula, weight, and available spectroscopic data for Tetrapropoxysilane?
A:
- Spectroscopic Data:
Q3: How do the properties of materials containing Tetrapropoxysilane change under different conditions, and what are some typical applications based on this behavior?
A3:
Q4: Does Tetrapropoxysilane exhibit catalytic properties? If so, what are the mechanisms and applications?
A4: While TPOS itself is not a catalyst, it plays a crucial role in supporting catalytic activity:
Q5: How is computational chemistry employed in research involving Tetrapropoxysilane?
A5: Computational methods provide insights into TPOS behavior:
- Reaction Mechanism: Simulations can elucidate the mechanism of TPOS hydrolysis and condensation, offering valuable information for controlling silica network formation. []
- Material Properties: Molecular dynamics simulations can predict the mechanical and thermal properties of hybrid materials containing TPOS, aiding in material design and optimization. []
Q6: How do modifications to the structure of Tetrapropoxysilane, such as changing the alkoxy group, influence its reactivity and applications?
A6: Modifying the alkoxy groups in tetraalkoxysilanes significantly impacts their properties:
Q7: How stable is Tetrapropoxysilane under various conditions, and what strategies can enhance its stability, solubility, or bioavailability?
A7: TPOS is sensitive to moisture and undergoes hydrolysis in the presence of water.
- Controlled Hydrolysis: The hydrolysis of TPOS can be controlled by adjusting the pH, temperature, and solvent composition. This control is crucial in applications like sol-gel processing to achieve desired material properties. [, ]
Q8: What are some significant historical milestones in the research and application of Tetrapropoxysilane?
A8: While specific historical milestones aren't explicitly mentioned in the provided abstracts, the research highlights TPOS's evolution within the field of material science. Key milestones likely include:
Q9: How does research on Tetrapropoxysilane intersect with other disciplines, leading to cross-disciplinary applications and collaborations?
A9: The research showcases how TPOS bridges various disciplines:
- Material Science & Engineering: TPOS plays a vital role in developing new materials with tailored properties. Collaborative efforts with material scientists and engineers drive innovation in areas like polymers, composites, and ceramics. [, , , , ]
- Chemistry & Chemical Engineering: Understanding the chemistry of TPOS, particularly its hydrolysis and condensation mechanisms, is crucial for controlling material synthesis and properties. This understanding fosters collaboration between chemists and chemical engineers to optimize reaction conditions and develop scalable production processes. [, , ]
- Nanotechnology & Nanoscience: The ability to synthesize mesoporous silica nanoparticles using TPOS opens doors for collaborative research in nanotechnology and nanoscience, impacting fields like drug delivery, catalysis, and sensing. [, , , ]
Disclaimer and Information on In-Vitro Research Products
Please be aware that all articles and product information presented on BenchChem are intended solely for informational purposes. The products available for purchase on BenchChem are specifically designed for in-vitro studies, which are conducted outside of living organisms. In-vitro studies, derived from the Latin term "in glass," involve experiments performed in controlled laboratory settings using cells or tissues. It is important to note that these products are not categorized as medicines or drugs, and they have not received approval from the FDA for the prevention, treatment, or cure of any medical condition, ailment, or disease. We must emphasize that any form of bodily introduction of these products into humans or animals is strictly prohibited by law. It is essential to adhere to these guidelines to ensure compliance with legal and ethical standards in research and experimentation.