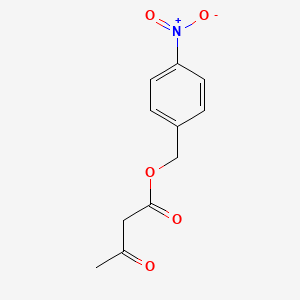
4-Nitrobenzyl acetoacetate
Overview
Description
4-Nitrobenzyl acetoacetate (CAS: 61312-84-3) is an organic ester with the molecular formula C₁₁H₁₁NO₅ and a molecular weight of 237.21 g/mol . Structurally, it consists of an acetoacetate backbone (CH₃C(O)CH₂C(O)O−) esterified with a 4-nitrobenzyl group, introducing strong electron-withdrawing properties due to the nitro substituent. The compound typically appears as a white to light yellow crystalline powder with a melting point of 44°C and a predicted boiling point of 376.5°C . Its density is approximately 1.287 g/cm³, and it is commonly stored under dry, room-temperature conditions to ensure stability .
This compound is widely used as a chemical building block in organic synthesis, particularly in the preparation of diazo compounds (e.g., 4-nitrobenzyl-2-diazoacetoacetate, a photoresist precursor) and as a substrate for catalytic reactions . Its high purity (>98% by HPLC) and reactivity make it valuable in pharmaceutical and materials science research .
Preparation Methods
Primary Synthetic Route: Esterification of 4-Nitrobenzyl Alcohol with Diketene Derivatives
Reaction Mechanism and Reagents
The most well-documented method for synthesizing 4-nitrobenzyl acetoacetate involves the reaction of 4-nitrobenzyl alcohol with 2,2-dimethyl-1,3-dioxane-4,6-dione (a cyclic diketene acetal) in toluene. This nucleophilic acyl substitution proceeds without catalysts, leveraging the electrophilicity of the diketene derivative. The reaction mechanism involves:
- Activation : The diketene’s carbonyl group is attacked by the hydroxyl oxygen of 4-nitrobenzyl alcohol.
- Intermediate Formation : A tetrahedral intermediate forms, followed by the expulsion of the leaving group (dimethyl carbonate analog).
- Ester Formation : The final product, this compound, precipitates upon cooling.
Optimization of Reaction Parameters
Temperature and Solvent Effects
The reaction is conducted at 30–35°C to balance reaction rate and product stability. Lower temperatures prolong the reaction, while higher temperatures risk side reactions (e.g., decomposition of the nitro group). Toluene is chosen for its ability to dissolve reactants while allowing product crystallization upon cooling.
Catalysts and Additives
No catalysts are required, as the diketene’s inherent reactivity drives the reaction. However, phase-transfer catalysts (e.g., tri-n-butyl methyl ammonium chloride) are used in subsequent diazotization steps, highlighting the compound’s utility in multi-step syntheses.
Characterization and Analytical Data
Physical Properties
Property | Value | Source |
---|---|---|
Melting Point | 42–46°C | |
Purity (HPLC) | >98.0% | |
Solubility | Methanol | |
Molecular Weight | 237.21 g/mol |
Spectroscopic Confirmation
- ¹H NMR : Peaks corresponding to the nitrobenzyl aromatic protons (δ 8.2–8.4 ppm), methylene groups (δ 3.4–3.6 ppm), and acetyl protons (δ 2.3 ppm).
- IR : Strong absorption at 1720 cm⁻¹ (ester carbonyl) and 1520 cm⁻¹ (nitro group).
Applications in Organic Synthesis
Preparation of Diazonium Compounds
This compound serves as a precursor for p-nitrobenzyl 2-diazoacetoacetate , a key intermediate in oligonucleotide modification. The diazotization step involves:
- Treating the ester with sodium azide and a phase-transfer catalyst.
- Generating the diazo group at the α-position of the ketone.
Functional Material Synthesis
The compound’s nitro group facilitates reduction-responsive applications, such as cleavable linkers in drug delivery systems.
Chemical Reactions Analysis
Transesterification Reactions
4-NBA participates in ester exchange reactions to form derivatives with modified biological or synthetic utility. A patented method (CN107556212B) demonstrates its synthesis via transesterification with ethyl 2-diazoacetoacetate under catalytic conditions :
Reaction Equation:
Optimized Conditions:
Parameter | Value |
---|---|
Catalyst | Sodium ethoxide (1 wt%) |
Solvent | Toluene/xylene |
Molar Ratio (Alcohol:Ester) | 1:1.2 |
Temperature | Reflux (~110°C) |
Yield | 94.2–96.5% |
This method avoids hazardous diazo reagents, offering a safer route to diazo derivatives for photolabile applications .
Photooxygenation and Acid-Catalyzed Hydrolysis
Under UV light in oxygenated aqueous media, 4-NBA undergoes photooxygenation via a carbanion intermediate, leading to oxidative cleavage products :
Mechanism Highlights:
-
Base-Catalyzed Pathway: Generates α-hydroperoxy ethers at pH >11.
-
Acid-Catalyzed Hydrolysis (pH <3): Produces nitrobenzaldehyde derivatives and hydrogen peroxide .
Example Reaction:
Key Observations:
-
Solvent isotope effects confirm water’s role as a proton donor/base .
-
No radical intermediates detected, ruling out free-radical pathways .
Reductive Cleavage
The nitro group and benzyl ester in 4-NBA enable reductive transformations:
Ester Cleavage
4-NBA’s benzyl ester undergoes reductive cleavage in biological systems. For example, diazo derivatives of 4-nitrobenzyl groups release active oligonucleotides upon reduction (e.g., using dithiothreitol) :
Comparative Reactivity
The table below contrasts 4-NBA’s reactivity with structurally similar esters:
Compound | Key Functional Groups | Reactivity Profile |
---|---|---|
4-NBA | Nitrobenzyl, acetoacetate | Photooxygenation, transesterification, reductive cleavage |
Benzyl acetate | Simple benzyl ester | Hydrolysis only under harsh conditions |
p-Nitrophenyl acetate | Nitrophenyl ester | Faster hydrolysis, no diazo coupling |
Scientific Research Applications
Applications in Organic Synthesis
1. Intermediate in Pharmaceutical Synthesis:
NBA serves as an important intermediate in the synthesis of various pharmaceuticals. Its structure allows for the introduction of a protected acetoacetate group onto other molecules, facilitating the creation of complex organic structures. The ability to cleave the benzyl ester group under mild conditions enhances its utility in synthetic pathways.
2. Synthesis of Derivatives:
Research indicates that derivatives of NBA can exhibit biological activities, including antibacterial and antifungal properties. For instance, certain esters derived from NBA have shown potential in inhibiting bacterial growth, making them candidates for further exploration in drug development .
Biochemical Research Applications
1. Enzyme Inhibition Studies:
NBA has been investigated for its potential to inhibit specific enzymes, which is crucial for developing therapeutic agents. Studies have shown that NBA derivatives can act as enzyme inhibitors, providing insights into their mechanism of action and potential therapeutic uses .
2. Interaction Studies:
Interaction studies involving NBA focus on its reactivity with various biological molecules. Understanding these interactions is essential for assessing how NBA can be effectively utilized in medicinal chemistry.
Mechanism of Action
The mechanism of action of 4-nitrobenzyl acetoacetate involves its interaction with specific molecular targets and pathways:
Comparison with Similar Compounds
Below is a detailed comparison of 4-nitrobenzyl acetoacetate with structurally analogous esters, focusing on molecular properties, reactivity, and applications.
Structural and Physical Properties
Compound Name | CAS Number | Molecular Formula | Molecular Weight (g/mol) | Melting Point (°C) | Boiling Point (°C) | Density (g/cm³) | Key Functional Groups |
---|---|---|---|---|---|---|---|
This compound | 61312-84-3 | C₁₁H₁₁NO₅ | 237.21 | 44 | 376.5 (predicted) | 1.287 | Acetoacetate, 4-nitrobenzyl |
Benzyl acetoacetate | 5396-89-4 | C₁₁H₁₂O₃ | 192.21 | N/A | 285 (lit.) | 1.112 | Acetoacetate, benzyl |
3-Pentyl acetoacetate | 13562-81-7 | C₉H₁₆O₃ | 172.22 | N/A | 230 (est.) | 0.983 | Acetoacetate, 3-pentyl |
Acetoacetic acid 2-methoxyethyl ester | 22502-03-0 | C₇H₁₂O₄ | 160.17 | N/A | 210 (est.) | 1.080 | Acetoacetate, 2-methoxyethyl |
Ethyl 4-nitrobenzoylacetate | N/A | C₁₁H₁₁NO₅ | 237.21 | 89–91 | N/A | 1.320 | Benzoylacetate, ethyl, 4-nitro |
Key Observations:
Electron-Withdrawing Effects: The 4-nitrobenzyl group in this compound enhances electrophilicity compared to non-nitro analogs like benzyl acetoacetate, making it more reactive in nucleophilic acyl substitution reactions .
Thermal Stability : The nitro group increases the compound’s melting point (44°C) relative to benzyl acetoacetate (liquid at room temperature) .
Solubility : The polar nitro group improves solubility in aprotic solvents (e.g., toluene, dichloromethane) compared to aliphatic esters like 3-pentyl acetoacetate .
Catalytic Reactions
This compound participates in Pechmann reactions and 1,4-dihydropyridine (1,4-DHP) syntheses when catalyzed by systems like Fe₃O₄@SiO₂@ADMPT/H₆P₂W₁₈O₆₂, achieving high yields (>90%) under mild conditions (50°C, solvent-free) . In contrast, ethyl acetoacetate—a non-nitro analog—requires higher temperatures (80–100°C) and longer reaction times for similar transformations .
Comparison with Ethyl 4-Nitrobenzoylacetate
While both compounds contain a nitro group, ethyl 4-nitrobenzoylacetate (CAS: N/A) features a benzoyl (C₆H₅C(O)−) instead of an acetoacetate moiety. This structural difference reduces its enol tautomerization capability, limiting its utility in keto-enol-based reactions .
Biological Activity
4-Nitrobenzyl acetoacetate (NBAA) is a synthetic compound that has garnered attention for its diverse biological activities. This article explores its pharmacological properties, mechanisms of action, and potential therapeutic applications based on various research findings.
Chemical Structure and Properties
This compound is an ester derived from acetoacetic acid and 4-nitrobenzyl alcohol. Its chemical structure can be represented as follows:
This compound features a nitro group, which is significant for its biological activity due to the electron-withdrawing nature of the nitro substituent.
1. Antimicrobial Activity
Research has indicated that NBAA exhibits notable antimicrobial properties. A study demonstrated its efficacy against various bacterial strains, including Escherichia coli and Staphylococcus aureus. The Minimum Inhibitory Concentration (MIC) values were determined, showcasing that NBAA can inhibit bacterial growth at relatively low concentrations.
Bacterial Strain | MIC (µg/mL) |
---|---|
E. coli | 32 |
Staphylococcus aureus | 16 |
These results suggest that NBAA could potentially serve as a lead compound for developing new antimicrobial agents.
2. Antifungal Activity
In addition to its antibacterial effects, NBAA has shown antifungal activity against strains such as Candida albicans. The study reported that NBAA inhibited fungal growth with an MIC value of 64 µg/mL, indicating moderate antifungal potential.
3. Anti-inflammatory Effects
NBAA's anti-inflammatory properties were evaluated in vitro using lipopolysaccharide (LPS)-stimulated macrophages. The compound significantly reduced nitric oxide (NO) production, a marker of inflammation, suggesting that it may inhibit the inflammatory response through the modulation of pro-inflammatory cytokines.
The biological activity of NBAA can be attributed to several mechanisms:
- Nitro Group Influence : The presence of the nitro group enhances the electron deficiency of the aromatic ring, which may facilitate interactions with various biological targets.
- Reactive Oxygen Species (ROS) Modulation : NBAA has been shown to influence ROS levels in cells, contributing to its protective effects against oxidative stress.
- Enzyme Inhibition : Preliminary studies suggest that NBAA may inhibit key enzymes involved in inflammatory pathways, although further research is needed to elucidate specific targets.
Case Studies and Research Findings
Several studies have explored the biological effects of NBAA:
- A study published in a peer-reviewed journal highlighted the compound's potential as a neuroprotective agent, demonstrating that it could protect retinal ganglion cells from excitotoxic damage induced by NMDA (N-methyl-D-aspartate) .
- Another investigation focused on the cytotoxic effects of NBAA on cancer cell lines such as HeLa and MCF-7. Results indicated that NBAA induces apoptosis in these cells, suggesting its potential as an anticancer agent.
Q & A
Basic Research Questions
Q. What are the optimal synthetic routes for preparing 4-nitrobenzyl acetoacetate, and how can reaction conditions be optimized?
- Methodology : A two-step transesterification process is commonly used. First, methyl acetoacetate reacts with 4-nitrobenzyl alcohol under reflux in anhydrous tetrahydrofuran (THF) with catalytic sodium hydride. The reaction is monitored via thin-layer chromatography (TLC) until completion. The product is purified via recrystallization from methanol, yielding this compound with >98% purity. Reaction optimization includes controlling temperature (reflux at ~66°C) and stoichiometric ratios (1:1.2 molar ratio of methyl acetoacetate to 4-nitrobenzyl alcohol) to minimize side products .
Q. How can researchers verify the purity and structural integrity of this compound?
- Methodology :
- Purity Analysis : Use HPLC with a C18 column (mobile phase: 70% methanol/30% water) to confirm ≥98% purity. Neutralization titration with sodium hydroxide can cross-validate acid-sensitive impurities .
- Structural Characterization : Employ H/C NMR (in CDCl₃) to identify ester carbonyl peaks (~170 ppm) and aromatic nitro groups. IR spectroscopy confirms C=O stretching (~1740 cm⁻¹) and NO₂ asymmetric stretching (~1520 cm⁻¹). High-resolution mass spectrometry (HRMS) validates the molecular ion peak (m/z 237.2087 for C₁₁H₁₁NO₅) .
Q. What solvent systems are recommended for dissolving this compound in experimental workflows?
- Methodology : The compound is highly soluble in methanol and dichloromethane (CH₂Cl₂) but sparingly soluble in water. For kinetic studies, use CH₂Cl₂ due to its low polarity and compatibility with UV-Vis spectroscopy (λₘₐₓ = 267 nm). For polar reaction environments, methanol is preferred, but ensure inert conditions to prevent ester hydrolysis .
Advanced Research Questions
Q. How does this compound participate in enzymatic decarboxylation mechanisms, and what structural insights are critical?
- Methodology : The compound serves as a substrate analog in studies of acetoacetate decarboxylase (AADase). X-ray crystallography (e.g., PDB ID 3BH2) reveals that the nitro group mimics the keto-enol tautomerization of acetoacetate. Key residues (Lys 115 and Lys 116) stabilize the transition state via electrostatic perturbations. Mutagenesis studies (e.g., K116A) can validate the role of microenvironment desolvation in lowering the pKₐ of catalytic lysine residues .
Q. How should researchers resolve discrepancies in purity metrics between HPLC and titration methods?
- Methodology : Discrepancies arise from non-UV-active impurities (e.g., inorganic salts) undetected by HPLC. Conduct parallel analyses:
HPLC : Quantifies organic impurities via area normalization.
Neutralization Titration : Detects acidic/basic contaminants (e.g., residual 4-nitrobenzyl alcohol).
Combine both methods and use mass balance calculations to adjust purification protocols (e.g., additional recrystallization) .
Q. What role does this compound play in metabolic pathway studies, particularly in ketone body analysis?
- Methodology : In metabolomics, the compound is a precursor for synthesizing deuterated internal standards. For example, in H NMR-based studies of cerebrospinal fluid, it aids in quantifying acetoacetate and β-hydroxybutyrate ratios. Use liquid chromatography-mass spectrometry (LC-MS) with a HILIC column to separate ketone bodies in biological matrices. Exclude creatinine and acetone during data normalization to avoid interference .
Q. How can molecular docking studies predict the interaction of this compound with acrylamide-based polymers?
- Methodology : Use AutoDock Vina to simulate binding affinities. Prepare the ligand (this compound) by optimizing its geometry with Gaussian09 at the B3LYP/6-31G* level. Dock into polymer models (e.g., PAM1-PAM5) using a grid box centered on the active site. Analyze binding poses for hydrogen bonding with amide groups and π-π stacking with aromatic moieties. Validate results via molecular dynamics simulations (100 ns) in GROMACS .
Q. What experimental strategies mitigate thermal instability during long-term storage of this compound?
- Methodology : Store at –20°C under argon in amber vials to prevent photodegradation. For lab-scale use, pre-dry solvents (e.g., molecular sieves in methanol) to avoid hydrolysis. Monitor decomposition via periodic HPLC checks. If degradation occurs (>2% over 6 months), repurify via flash chromatography (silica gel, hexane/ethyl acetate 7:3) .
Q. Data Contradiction Analysis
Q. How should conflicting data on melting points (42–46°C vs. 44°C) be addressed?
Properties
IUPAC Name |
(4-nitrophenyl)methyl 3-oxobutanoate | |
---|---|---|
Source | PubChem | |
URL | https://pubchem.ncbi.nlm.nih.gov | |
Description | Data deposited in or computed by PubChem | |
InChI |
InChI=1S/C11H11NO5/c1-8(13)6-11(14)17-7-9-2-4-10(5-3-9)12(15)16/h2-5H,6-7H2,1H3 | |
Source | PubChem | |
URL | https://pubchem.ncbi.nlm.nih.gov | |
Description | Data deposited in or computed by PubChem | |
InChI Key |
KQPGVCMZXFBYMM-UHFFFAOYSA-N | |
Source | PubChem | |
URL | https://pubchem.ncbi.nlm.nih.gov | |
Description | Data deposited in or computed by PubChem | |
Canonical SMILES |
CC(=O)CC(=O)OCC1=CC=C(C=C1)[N+](=O)[O-] | |
Source | PubChem | |
URL | https://pubchem.ncbi.nlm.nih.gov | |
Description | Data deposited in or computed by PubChem | |
Molecular Formula |
C11H11NO5 | |
Source | PubChem | |
URL | https://pubchem.ncbi.nlm.nih.gov | |
Description | Data deposited in or computed by PubChem | |
DSSTOX Substance ID |
DTXSID90375411 | |
Record name | (4-nitrophenyl)methyl 3-oxobutanoate | |
Source | EPA DSSTox | |
URL | https://comptox.epa.gov/dashboard/DTXSID90375411 | |
Description | DSSTox provides a high quality public chemistry resource for supporting improved predictive toxicology. | |
Molecular Weight |
237.21 g/mol | |
Source | PubChem | |
URL | https://pubchem.ncbi.nlm.nih.gov | |
Description | Data deposited in or computed by PubChem | |
CAS No. |
61312-84-3 | |
Record name | Butanoic acid, 3-oxo-, (4-nitrophenyl)methyl ester | |
Source | CAS Common Chemistry | |
URL | https://commonchemistry.cas.org/detail?cas_rn=61312-84-3 | |
Description | CAS Common Chemistry is an open community resource for accessing chemical information. Nearly 500,000 chemical substances from CAS REGISTRY cover areas of community interest, including common and frequently regulated chemicals, and those relevant to high school and undergraduate chemistry classes. This chemical information, curated by our expert scientists, is provided in alignment with our mission as a division of the American Chemical Society. | |
Explanation | The data from CAS Common Chemistry is provided under a CC-BY-NC 4.0 license, unless otherwise stated. | |
Record name | (4-nitrophenyl)methyl 3-oxobutanoate | |
Source | EPA DSSTox | |
URL | https://comptox.epa.gov/dashboard/DTXSID90375411 | |
Description | DSSTox provides a high quality public chemistry resource for supporting improved predictive toxicology. | |
Record name | Butanoic acid, 3-oxo-, (4-nitrophenyl)methyl ester | |
Source | European Chemicals Agency (ECHA) | |
URL | https://echa.europa.eu/substance-information/-/substanceinfo/100.112.661 | |
Description | The European Chemicals Agency (ECHA) is an agency of the European Union which is the driving force among regulatory authorities in implementing the EU's groundbreaking chemicals legislation for the benefit of human health and the environment as well as for innovation and competitiveness. | |
Explanation | Use of the information, documents and data from the ECHA website is subject to the terms and conditions of this Legal Notice, and subject to other binding limitations provided for under applicable law, the information, documents and data made available on the ECHA website may be reproduced, distributed and/or used, totally or in part, for non-commercial purposes provided that ECHA is acknowledged as the source: "Source: European Chemicals Agency, http://echa.europa.eu/". Such acknowledgement must be included in each copy of the material. ECHA permits and encourages organisations and individuals to create links to the ECHA website under the following cumulative conditions: Links can only be made to webpages that provide a link to the Legal Notice page. | |
Synthesis routes and methods
Procedure details
Retrosynthesis Analysis
AI-Powered Synthesis Planning: Our tool employs the Template_relevance Pistachio, Template_relevance Bkms_metabolic, Template_relevance Pistachio_ringbreaker, Template_relevance Reaxys, Template_relevance Reaxys_biocatalysis model, leveraging a vast database of chemical reactions to predict feasible synthetic routes.
One-Step Synthesis Focus: Specifically designed for one-step synthesis, it provides concise and direct routes for your target compounds, streamlining the synthesis process.
Accurate Predictions: Utilizing the extensive PISTACHIO, BKMS_METABOLIC, PISTACHIO_RINGBREAKER, REAXYS, REAXYS_BIOCATALYSIS database, our tool offers high-accuracy predictions, reflecting the latest in chemical research and data.
Strategy Settings
Precursor scoring | Relevance Heuristic |
---|---|
Min. plausibility | 0.01 |
Model | Template_relevance |
Template Set | Pistachio/Bkms_metabolic/Pistachio_ringbreaker/Reaxys/Reaxys_biocatalysis |
Top-N result to add to graph | 6 |
Feasible Synthetic Routes
Disclaimer and Information on In-Vitro Research Products
Please be aware that all articles and product information presented on BenchChem are intended solely for informational purposes. The products available for purchase on BenchChem are specifically designed for in-vitro studies, which are conducted outside of living organisms. In-vitro studies, derived from the Latin term "in glass," involve experiments performed in controlled laboratory settings using cells or tissues. It is important to note that these products are not categorized as medicines or drugs, and they have not received approval from the FDA for the prevention, treatment, or cure of any medical condition, ailment, or disease. We must emphasize that any form of bodily introduction of these products into humans or animals is strictly prohibited by law. It is essential to adhere to these guidelines to ensure compliance with legal and ethical standards in research and experimentation.