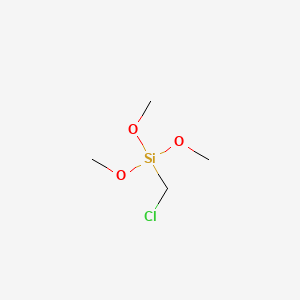
(Chloromethyl)trimethoxysilane
Overview
Description
(Chloromethyl)trimethoxysilane (CMTMS) is a bifunctional organosilane with the molecular formula C₄H₁₁ClO₃Si. Its structure consists of a reactive chloromethyl (-CH₂Cl) group attached to a trimethoxysilane (-Si(OCH₃)₃) moiety. The chloromethyl group acts as a leaving group, enabling nucleophilic substitution reactions, while the trimethoxysilane facilitates hydrolysis and condensation with hydroxylated surfaces (e.g., glass, metals, or polymers) . This dual functionality makes CMTMS a versatile coupling agent in:
Preparation Methods
Industrial-Scale Synthesis via Direct Esterification
The predominant industrial method involves esterification of Chloromethyltrichlorosilane (CMTCS) with methanol under controlled conditions. Key parameters from CN105646564B include:
Reaction Setup and Conditions
- Reactants : CMTCS and absolute methanol in a 1:3.1–3.3 molar ratio.
- Catalyst : None required due to methanol’s nucleophilic properties.
- Temperature : Initial heating to 118–120°C to initiate reflux, followed by gradual temperature escalation to 140–155°C during methanol addition.
- Neutralization : Post-reaction treatment with triethylamine or tri-n-butylamine (0.02–0.05% of CMTCS mass) adjusts pH to 7–8, removing HCl byproducts.
Process Optimization
- Methanol Addition Rate : Controlled over 6.0–6.5 hours to prevent runaway reactions.
- Distillation : Vacuum distillation (60–80°C, 10–20 mmHg) yields >98% purity product.
- Yield : 96–99.5% achieved through precise temperature modulation and neutralization.
Table 1: Industrial Synthesis Performance Metrics
Parameter | Example 1 | Example 2 | Example 3 |
---|---|---|---|
Methanol Feed Time (h) | 6.0 | 6.5 | 6.5 |
Final Temp (°C) | 140 | 145 | 155 |
Product Yield (%) | 97.2 | 98.8 | 99.5 |
Purity (GC, %) | 98.2 | 98.9 | 99.2 |
Cl⁻ Content (ppm) | 21 | 7 | 3 |
Alternative Two-Step Alkoxylation
CN106632449A describes a sodium alkoxide-mediated approach adaptable for CMMS:
Step 1: Chloromethyltrialkoxysilane Synthesis
- Reagents : CMTCS reacts with sodium methoxide (NaOCH₃) in methanol (4:1 molar ratio).
- Conditions : Dropwise addition at 1 drop/sec under N₂, followed by 30-min room-temperature reaction.
- Workup : Atmospheric distillation removes methanol; filtration eliminates NaCl byproducts.
Step 2: Amine Quaternization (Adaptable for Methoxy Systems)
- Reagents : Intermediate product reacts with amines (e.g., trioctylamine) at 70–200°C.
- Yield : >90% after vacuum distillation, though methoxy systems require tighter temperature control than ethoxy analogs.
Sol-Gel Derivatization for Functional Materials
Recent advances exploit CMMS’s reactivity in hybrid material synthesis:
Aerogel Fabrication
- Procedure : Hydrolysis of CMMS in acidic ethanol/water mixtures forms polychloromethylsilsesquioxane (PCMSQ) colloids.
- Functionalization : SN2 reactions with polyamines (e.g., polyethyleneimine) crosslink siloxane networks, enhancing mechanical strength.
- Applications : Porous supports for carbon dots (C-dots) in photoluminescent composites.
Quality Control and Analytical Characterization
Purity Assessment
- Gas Chromatography (GC) : Quantifies CMMS content (>98%) and detects residual methanol (<1.5%).
- Chloride Ion Analysis : Potentiometric titration ensures Cl⁻ levels <50 ppm for corrosion-sensitive applications.
Structural Verification
Emerging Trends and Challenges
Continuous-Flow Reactors
- Benefits : Improved heat distribution reduces byproducts (e.g., tetramethoxysilane).
- Limitations : Catalyst fouling in high-viscosity systems.
Green Chemistry Initiatives
Chemical Reactions Analysis
Hydrolysis and Condensation Reactions
The compound undergoes hydrolysis in the presence of water, forming silanols and methanol. These silanols further condense to form siloxane bonds, a critical process in sol-gel chemistry .
Key Observations:
-
Hydrolysis Conditions :
-
Acidic or basic catalysis accelerates hydrolysis.
-
Silanol intermediates are unstable and prone to condensation.
-
-
Condensation Products :
Table 1: Hydrolysis and Condensation Behavior
Reaction Type | Conditions | Products |
---|---|---|
Hydrolysis | Acidic/Basic | Silanols + CH₃OH |
Condensation | Neutral/Acidic | Siloxane polymers |
Substitution Reactions
The chloromethyl group undergoes nucleophilic substitution , reacting with nucleophiles such as amines or alcohols.
Quaternization with Amines
(Chloromethyl)trimethoxysilane reacts with tertiary amines (e.g., trioctylamine) to form quaternary ammonium salts , which are used in separation materials .
Mechanism :
-
Nucleophilic attack by amine on the chloromethyl group.
-
Formation of a quaternary ammonium salt after chloride elimination.
Table 2: Substitution with Trioctylamine
Reagent | Conditions | Product |
---|---|---|
Trioctylamine | Room temperature, stirring | Quaternary ammonium salt |
Coupling Reactions
The compound participates in carboxylative coupling reactions, such as the Hiyama coupling, under palladium catalysis.
Carboxylative Hiyama Coupling
Reaction with allyltrimethoxysilane and CO₂ produces α,β-unsaturated esters .
Mechanism :
-
Formation of a π-allylpalladium chloride intermediate.
-
Insertion of CO₂ and subsequent coupling with the silane.
Table 3: Carboxylative Coupling Details
Catalyst | Reagents | Products | Yields |
---|---|---|---|
Pd nanoparticles | Allyltrimethoxysilane, CO₂ | α,β-unsaturated esters | 55–85% |
Scientific Research Applications
Surface Modification
CMTMS is extensively utilized for modifying surfaces of inorganic materials such as glass, silica, and metal oxides. The modification enhances adhesion properties and introduces functional groups that can facilitate further chemical reactions or biological interactions.
Biological Applications
In biological contexts, CMTMS has been employed for:
- Drug Delivery Systems : Surface modification of silica nanoparticles with CMTMS improves drug loading and release profiles, enhancing therapeutic efficacy.
- Bioconjugation : The chloromethyl group allows for stable bonding with thiol-containing biomolecules, facilitating the development of biosensors and targeted drug delivery systems.
Materials Science
CMTMS plays a pivotal role in the synthesis of advanced materials:
- Sol-Gel Processing : It acts as a coupling agent during sol-gel transitions, contributing to the formation of silica-based networks with tailored properties.
- Aerogels and Mesoporous Silica Gels : CMTMS is used in producing aerogels due to its ability to create porous structures with high surface areas suitable for various applications including catalysis and adsorption.
Environmental Applications
Recent studies have explored the use of CMTMS-modified materials for environmental remediation, particularly in adsorbing heavy metals from wastewater . The functionalization enhances the materials' capacity to sequester pollutants effectively.
Case Study 1: Drug Delivery Systems
A study demonstrated that silica nanoparticles modified with CMTMS exhibited improved stability and controlled release characteristics for anticancer drugs. The incorporation of CMTMS facilitated better interaction between the drug and the carrier material, resulting in enhanced therapeutic outcomes.
Case Study 2: Heavy Metal Adsorption
Research focusing on CMTMS-modified silica gel showed significant improvements in adsorption capacities for Th(IV) ions from aqueous solutions. Characterization techniques such as FTIR and BET were employed to analyze the functionalization process and adsorption kinetics, confirming the effectiveness of CMTMS in environmental applications .
Mechanism of Action
The mechanism of action of (Chloromethyl)trimethoxysilane involves the hydrolysis of the methoxy groups to form silanols, which can then condense to form siloxane bonds. The chloromethyl group can participate in nucleophilic substitution reactions, allowing for the functionalization of surfaces and the formation of various silane derivatives .
Comparison with Similar Compounds
Comparison with Similar Organosilanes
Structural and Functional Differences
Reactivity and Application-Specific Performance
Hydrolysis and Condensation
- CMTMS hydrolyzes faster than ethoxy-based analogs (e.g., chloromethyltriethoxysilane) due to the higher electrophilicity of methoxy groups, enabling rapid sol-gel network formation .
- CPETS’s aromatic spacer slows hydrolysis but enhances thermal stability in crosslinked membranes .
Quaternization and Ion Exchange
- CMTMS reacts with amines (e.g., tris-(2-hydroxyethyl)amine) to form quaternary ammonium salts in near-quantitative yields . This is critical for synthesizing anion exchange membranes with high ion capacity .
- In contrast, pre-quaternized silanes (e.g., (3-N,N,N-triethanolammoniopropyl)trimethoxysilane chloride) exhibit immediate antibacterial effects without requiring additional modification .
Surface Modification
- CMTMS-modified glass or silica shows improved adhesion for coatings but lacks inherent antimicrobial activity .
- Quaternary ammonium silanes reduce bacterial adhesion by >3 orders of magnitude, outperforming CMTMS in biomedical applications .
Chromatography
- 4-(Chloromethyl)phenyltrimethoxysilane’s phenyl group enhances hydrophobicity in HILIC columns, improving separation of sugars and polar compounds . CMTMS, lacking an aromatic spacer, is less effective in such setups.
Key Research Findings
Membrane Performance
- PBI-Ph Membranes (CPETS-crosslinked) : Achieved proton conductivity of 0.083 S/cm at 160°C, outperforming CMTMS-based membranes (0.065 S/cm) due to rigid aromatic networks .
- CMTMS-Quaternized Polysulfone : Exhibited 1.8 mmol/g ion exchange capacity and 12.5 mS/cm conductivity, suitable for fuel cells .
Antibacterial Activity
- Quaternary ammonium silanes reduced Aeromonas hydrophila adhesion by 99.9%, while CMTMS required post-functionalization to achieve similar results .
Chromatography
- Columns with 4-(chloromethyl)phenyltrimethoxysilane spacers showed 15% higher efficiency in separating maltose anomers than CMTMS-derived phases .
Biological Activity
(Chloromethyl)trimethoxysilane (CMTMS) is a trialkoxysilane compound notable for its diverse applications in materials science and potential biological activities. This article explores its biological activity, synthesis, and relevant research findings, including data tables and case studies.
Chemical Structure and Properties
CMTMS has the chemical formula and is characterized by the presence of chloromethyl and trimethoxy functional groups. The chloromethyl group can react with various nucleophiles, making CMTMS useful for functionalizing surfaces and creating hybrid materials.
Synthesis of this compound
The synthesis of CMTMS typically involves the reaction of chloromethyltrichlorosilane with methanol, yielding a high-purity product. This method has been optimized to achieve yields above 96% with minimal byproducts, primarily hydrogen chloride .
Toxicity and Carcinogenic Potential
Research indicates that chloromethyl compounds, including CMTMS, may exhibit genotoxicity and potential carcinogenic effects. For instance, studies on chloromethyl methyl ether (a related compound) have shown associations with lung cancer among exposed workers, suggesting a similar risk profile for CMTMS due to its structural similarities .
- Case Studies:
- In a cohort study of workers exposed to chloromethyl methyl ether, a significant increase in lung cancer cases was documented, with a relative risk of 10.97 for developing lung cancer among those exposed .
- Animal studies have also indicated tumorigenic potential in various species when exposed to chloromethyl compounds .
Biocompatibility and Applications
Despite concerns regarding toxicity, CMTMS has been investigated for its potential use in biocompatible materials. Its ability to modify surfaces can enhance the adhesion of biomolecules, making it suitable for applications in drug delivery systems and tissue engineering.
- Research Findings:
- A study explored the use of silicon-based quaternary ammonium materials derived from CMTMS as adsorbents for heavy metals, indicating its utility in environmental applications .
- The modification of silica nanoparticles with CMTMS has shown promise in improving drug delivery efficiency by enhancing the stability and release profiles of therapeutic agents .
Data Tables
Study | Compound | Findings | Outcome |
---|---|---|---|
Figueroa et al., 1973 | Chloromethyl methyl ether | Increased lung cancer incidence among workers | High risk associated with exposure |
Maher & DeFonso, 1987 | Chloromethyl methyl ether | Mortality from respiratory cancer increased significantly | Long latency period observed |
Research on CMTMS | CMTMS derivatives | Enhanced adsorption properties for heavy metals | Potential environmental application |
Q & A
Basic Research Questions
Q. What are the standard synthetic routes for (Chloromethyl)trimethoxysilane, and how is its purity validated in academic research?
this compound is typically synthesized via nucleophilic substitution reactions between chloromethyl precursors (e.g., chloromethyl chloride) and trimethoxysilane derivatives under inert atmospheres. Solvents like toluene or THF are used to facilitate the reaction, often catalyzed by bases such as triethylamine to neutralize HCl byproducts . Methodological validation :
- Purity analysis : Gas chromatography (GC) with flame ionization detection is standard, as commercial batches often report >95% purity .
- Structural confirmation : and NMR spectroscopy identify characteristic peaks (e.g., Si-OCH at ~3.5 ppm, CHCl at ~4.8 ppm). FTIR confirms Si-O (1050–1100 cm) and C-Cl (600–800 cm) bonds .
Q. How is this compound used in surface modification of silica-based materials?
This compound acts as a silane coupling agent, enabling covalent bonding between silica surfaces and organic polymers. Key steps include:
Substrate pretreatment : Silica is activated via acid/base treatment to generate surface hydroxyl groups.
Grafting : Hydrolysis of methoxy groups forms silanol (-Si-OH), which condenses with silica surface -OH groups. The chloromethyl moiety facilitates subsequent nucleophilic reactions (e.g., quaternization or polymer grafting) .
Critical factors :
- Solvent polarity : Polar solvents (e.g., water/ethanol mixtures) enhance hydrolysis rates.
- Reaction time/temperature : Longer durations (12–24 hrs) at 60–80°C improve grafting density .
Advanced Research Questions
Q. What strategies optimize this compound’s role in synthesizing hybrid catalysts for C–C coupling reactions?
Advanced applications involve immobilizing metal catalysts (e.g., Pd) on silica supports functionalized with this compound. A representative method:
Co-condensation : Mix tetraethylorthosilicate (TEOS) and this compound with structure-directing agents (e.g., CTAB) to form mesoporous silica.
Metal coordination : The chloromethyl group reacts with amine ligands (e.g., 2-aminopyridine), which chelate Pd(II) ions.
Catalytic performance : The hybrid catalyst achieves >90% yield in water-medium Suzuki-Miyaura reactions and retains activity over 5 cycles due to stable Si-C bonds .
Key variables :
- Pore size : Adjustable via CTAB concentration to control substrate diffusion.
- Ligand density : Higher silane loading increases active sites but may reduce surface area .
Q. How do competing side reactions during this compound functionalization impact material design?
The chloromethyl group’s reactivity can lead to unintended crosslinking or hydrolysis:
- Hydrolysis vs. condensation : Excess water promotes premature silanol condensation, reducing grafting efficiency. Controlled humidity (<40%) is critical .
- Nucleophilic substitution : Competing reactions with solvents (e.g., ethanol) may form ethoxy derivatives. Anhydrous conditions and inert atmospheres mitigate this .
Analytical tools : - TGA/DSC : Quantify organic content and thermal stability of grafted materials.
- XPS : Confirm Si-C bonding and detect chloride residues from incomplete reactions .
Q. What are the emerging applications of this compound in stimuli-responsive nanomaterials?
Recent studies exploit its dual functionality (silane + chloromethyl) to design pH- or redox-responsive carriers:
- Drug delivery : Chloromethyl groups react with thiols in glutathione-rich environments (e.g., cancer cells), triggering payload release.
- Nanocomposite synthesis : Grafting onto magnetic silica nanoparticles enables reversible aggregation under magnetic fields .
Challenges : - Biocompatibility : Residual chloride may require post-synthesis quenching (e.g., with amines).
- Stability : Long-term storage in humid conditions risks siloxane network degradation .
Q. Safety and Handling
Q. What safety protocols are essential for handling this compound in academic labs?
- PPE : Wear nitrile gloves, safety goggles, and lab coats. Use in fume hoods with sash height <18 inches .
- Storage : Keep in airtight containers under nitrogen, away from moisture and ignition sources. Refrigerate at 2–8°C for long-term stability .
- Spill management : Absorb with vermiculite, neutralize with sodium bicarbonate, and dispose as hazardous waste .
Note : The compound is hygroscopic and may emit HCl upon hydrolysis—monitor pH during reactions .
Properties
IUPAC Name |
chloromethyl(trimethoxy)silane | |
---|---|---|
Source | PubChem | |
URL | https://pubchem.ncbi.nlm.nih.gov | |
Description | Data deposited in or computed by PubChem | |
InChI |
InChI=1S/C4H11ClO3Si/c1-6-9(4-5,7-2)8-3/h4H2,1-3H3 | |
Source | PubChem | |
URL | https://pubchem.ncbi.nlm.nih.gov | |
Description | Data deposited in or computed by PubChem | |
InChI Key |
FPOSCXQHGOVVPD-UHFFFAOYSA-N | |
Source | PubChem | |
URL | https://pubchem.ncbi.nlm.nih.gov | |
Description | Data deposited in or computed by PubChem | |
Canonical SMILES |
CO[Si](CCl)(OC)OC | |
Source | PubChem | |
URL | https://pubchem.ncbi.nlm.nih.gov | |
Description | Data deposited in or computed by PubChem | |
Molecular Formula |
C4H11ClO3Si | |
Source | PubChem | |
URL | https://pubchem.ncbi.nlm.nih.gov | |
Description | Data deposited in or computed by PubChem | |
DSSTOX Substance ID |
DTXSID10207987 | |
Record name | Silane, chloromethyl-trimethoxy- | |
Source | EPA DSSTox | |
URL | https://comptox.epa.gov/dashboard/DTXSID10207987 | |
Description | DSSTox provides a high quality public chemistry resource for supporting improved predictive toxicology. | |
Molecular Weight |
170.66 g/mol | |
Source | PubChem | |
URL | https://pubchem.ncbi.nlm.nih.gov | |
Description | Data deposited in or computed by PubChem | |
CAS No. |
5926-26-1 | |
Record name | (Chloromethyl)trimethoxysilane | |
Source | CAS Common Chemistry | |
URL | https://commonchemistry.cas.org/detail?cas_rn=5926-26-1 | |
Description | CAS Common Chemistry is an open community resource for accessing chemical information. Nearly 500,000 chemical substances from CAS REGISTRY cover areas of community interest, including common and frequently regulated chemicals, and those relevant to high school and undergraduate chemistry classes. This chemical information, curated by our expert scientists, is provided in alignment with our mission as a division of the American Chemical Society. | |
Explanation | The data from CAS Common Chemistry is provided under a CC-BY-NC 4.0 license, unless otherwise stated. | |
Record name | Silane, chloromethyl-trimethoxy- | |
Source | ChemIDplus | |
URL | https://pubchem.ncbi.nlm.nih.gov/substance/?source=chemidplus&sourceid=0005926261 | |
Description | ChemIDplus is a free, web search system that provides access to the structure and nomenclature authority files used for the identification of chemical substances cited in National Library of Medicine (NLM) databases, including the TOXNET system. | |
Record name | Silane, chloromethyl-trimethoxy- | |
Source | EPA DSSTox | |
URL | https://comptox.epa.gov/dashboard/DTXSID10207987 | |
Description | DSSTox provides a high quality public chemistry resource for supporting improved predictive toxicology. | |
Record name | (Chloromethyl)trimethoxysilane | |
Source | European Chemicals Agency (ECHA) | |
URL | https://echa.europa.eu/substance-information/-/substanceinfo/100.131.417 | |
Description | The European Chemicals Agency (ECHA) is an agency of the European Union which is the driving force among regulatory authorities in implementing the EU's groundbreaking chemicals legislation for the benefit of human health and the environment as well as for innovation and competitiveness. | |
Explanation | Use of the information, documents and data from the ECHA website is subject to the terms and conditions of this Legal Notice, and subject to other binding limitations provided for under applicable law, the information, documents and data made available on the ECHA website may be reproduced, distributed and/or used, totally or in part, for non-commercial purposes provided that ECHA is acknowledged as the source: "Source: European Chemicals Agency, http://echa.europa.eu/". Such acknowledgement must be included in each copy of the material. ECHA permits and encourages organisations and individuals to create links to the ECHA website under the following cumulative conditions: Links can only be made to webpages that provide a link to the Legal Notice page. | |
Retrosynthesis Analysis
AI-Powered Synthesis Planning: Our tool employs the Template_relevance Pistachio, Template_relevance Bkms_metabolic, Template_relevance Pistachio_ringbreaker, Template_relevance Reaxys, Template_relevance Reaxys_biocatalysis model, leveraging a vast database of chemical reactions to predict feasible synthetic routes.
One-Step Synthesis Focus: Specifically designed for one-step synthesis, it provides concise and direct routes for your target compounds, streamlining the synthesis process.
Accurate Predictions: Utilizing the extensive PISTACHIO, BKMS_METABOLIC, PISTACHIO_RINGBREAKER, REAXYS, REAXYS_BIOCATALYSIS database, our tool offers high-accuracy predictions, reflecting the latest in chemical research and data.
Strategy Settings
Precursor scoring | Relevance Heuristic |
---|---|
Min. plausibility | 0.01 |
Model | Template_relevance |
Template Set | Pistachio/Bkms_metabolic/Pistachio_ringbreaker/Reaxys/Reaxys_biocatalysis |
Top-N result to add to graph | 6 |
Feasible Synthetic Routes
Disclaimer and Information on In-Vitro Research Products
Please be aware that all articles and product information presented on BenchChem are intended solely for informational purposes. The products available for purchase on BenchChem are specifically designed for in-vitro studies, which are conducted outside of living organisms. In-vitro studies, derived from the Latin term "in glass," involve experiments performed in controlled laboratory settings using cells or tissues. It is important to note that these products are not categorized as medicines or drugs, and they have not received approval from the FDA for the prevention, treatment, or cure of any medical condition, ailment, or disease. We must emphasize that any form of bodily introduction of these products into humans or animals is strictly prohibited by law. It is essential to adhere to these guidelines to ensure compliance with legal and ethical standards in research and experimentation.