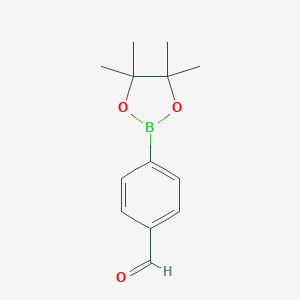
4-(4,4,5,5-Tetramethyl-1,3,2-dioxaborolan-2-yl)benzaldehyde
Overview
Description
“4-(4,4,5,5-Tetramethyl-1,3,2-dioxaborolan-2-yl)benzaldehyde” is a chemical compound with the formula C12H17BO2 . It is also known as phenylboronic acid pinacol ester . At room temperature, it appears as a colorless oily substance . It is commonly used in the preparation of pharmaceuticals and chemical intermediates .
Synthesis Analysis
The synthesis of “this compound” involves borylation at the benzylic C-H bond of alkylbenzenes in the presence of a palladium catalyst to form pinacol benzyl boronate . Hydroboration of alkyl or aryl alkynes and alkenes also occurs in the presence of transition metal catalysts .Molecular Structure Analysis
The molecular structure of “this compound” consists of 12 carbon atoms, 17 hydrogen atoms, 1 boron atom, and 2 oxygen atoms .Chemical Reactions Analysis
The chemical reactions involving “this compound” include borylation of arenes and the preparation of fluorenylborolane . It can also be used in the synthesis of intermediates for generating conjugated copolymers .Physical And Chemical Properties Analysis
“this compound” is a colorless oily substance at room temperature . Its molecular formula is C12H17BO2 .Scientific Research Applications
Synthesis and Structural Analysis
- 4-(4,4,5,5-Tetramethyl-1,3,2-dioxaborolan-2-yl)benzaldehyde and related compounds are used in synthesis and crystal structure studies. These compounds are obtained through substitution reactions and analyzed using techniques like FTIR, NMR spectroscopy, mass spectrometry, and X-ray diffraction. Their molecular structures are further validated through density functional theory (DFT) calculations (Huang et al., 2021).
Chemical Reactions and Properties
- This chemical is involved in the synthesis of various organic compounds, including boric acid ester intermediates with benzene rings. These syntheses involve complex chemical reactions, and the resulting compounds' properties are studied for potential applications in different fields (Huang et al., 2021).
Catalysis and Chemical Transformations
- It is used in palladium-catalyzed borylation reactions. These reactions are crucial in synthesizing aryl compounds and studying their properties, such as molecular electrostatic potential and frontier molecular orbitals (Takagi & Yamakawa, 2013).
Detection and Sensing Applications
- Derivatives of this compound have been studied for their potential in detecting substances like hydrogen peroxide vapor. This is particularly relevant in the context of explosive detection and environmental monitoring (Fu et al., 2016).
Pharmaceutical and Biological Research
- It has been investigated in the context of antifungal and antibacterial bioactivity. Certain derivatives show significant activity against fungi like Aspergillus niger and bacteria like Bacillus cereus, indicating potential pharmaceutical applications (Irving et al., 2003).
Material Science and Polymer Chemistry
- This compound is utilized in the synthesis of electronic materials, especially in the context of semiconductor technology. Its derivatives are instrumental in developing new materials with specific electronic properties (Zhu et al., 2007).
Mechanism of Action
Target of Action
It’s known that this compound is often used in organic synthesis, particularly in suzuki-miyaura coupling reactions .
Mode of Action
In the context of organic synthesis, 4-(4,4,5,5-Tetramethyl-1,3,2-dioxaborolan-2-yl)benzaldehyde can act as a boron source in palladium-catalyzed cross-coupling reactions . The boron atom in the compound can form a covalent bond with a carbon atom in another molecule, facilitating the formation of complex organic structures.
Biochemical Pathways
The compound is primarily involved in the Suzuki-Miyaura cross-coupling reaction pathway . This reaction is a type of palladium-catalyzed cross-coupling reaction, which is used to synthesize biaryl compounds—one of the most important structural motifs in pharmaceuticals and materials science.
Action Environment
The efficacy and stability of this compound, like many other organic compounds, can be influenced by various environmental factors. These include temperature, pH, and the presence of a catalyst. For instance, the compound is typically stored under inert gas at room temperature .
: TCI chemicals product page : Sigma Aldrich product page
Safety and Hazards
Future Directions
properties
IUPAC Name |
4-(4,4,5,5-tetramethyl-1,3,2-dioxaborolan-2-yl)benzaldehyde | |
---|---|---|
Source | PubChem | |
URL | https://pubchem.ncbi.nlm.nih.gov | |
Description | Data deposited in or computed by PubChem | |
InChI |
InChI=1S/C13H17BO3/c1-12(2)13(3,4)17-14(16-12)11-7-5-10(9-15)6-8-11/h5-9H,1-4H3 | |
Source | PubChem | |
URL | https://pubchem.ncbi.nlm.nih.gov | |
Description | Data deposited in or computed by PubChem | |
InChI Key |
DMBMXJJGPXADPO-UHFFFAOYSA-N | |
Source | PubChem | |
URL | https://pubchem.ncbi.nlm.nih.gov | |
Description | Data deposited in or computed by PubChem | |
Canonical SMILES |
B1(OC(C(O1)(C)C)(C)C)C2=CC=C(C=C2)C=O | |
Source | PubChem | |
URL | https://pubchem.ncbi.nlm.nih.gov | |
Description | Data deposited in or computed by PubChem | |
Molecular Formula |
C13H17BO3 | |
Source | PubChem | |
URL | https://pubchem.ncbi.nlm.nih.gov | |
Description | Data deposited in or computed by PubChem | |
DSSTOX Substance ID |
DTXSID50377754 | |
Record name | 4-(4,4,5,5-Tetramethyl-1,3,2-dioxaborolan-2-yl)benzaldehyde | |
Source | EPA DSSTox | |
URL | https://comptox.epa.gov/dashboard/DTXSID50377754 | |
Description | DSSTox provides a high quality public chemistry resource for supporting improved predictive toxicology. | |
Molecular Weight |
232.09 g/mol | |
Source | PubChem | |
URL | https://pubchem.ncbi.nlm.nih.gov | |
Description | Data deposited in or computed by PubChem | |
CAS RN |
128376-64-7 | |
Record name | 4-(4,4,5,5-Tetramethyl-1,3,2-dioxaborolan-2-yl)benzaldehyde | |
Source | EPA DSSTox | |
URL | https://comptox.epa.gov/dashboard/DTXSID50377754 | |
Description | DSSTox provides a high quality public chemistry resource for supporting improved predictive toxicology. | |
Record name | 4-(4,4,5,5-Tetramethyl-1,3,2-dioxaborolan-2-yl)benzaldehyde | |
Source | European Chemicals Agency (ECHA) | |
URL | https://echa.europa.eu/information-on-chemicals | |
Description | The European Chemicals Agency (ECHA) is an agency of the European Union which is the driving force among regulatory authorities in implementing the EU's groundbreaking chemicals legislation for the benefit of human health and the environment as well as for innovation and competitiveness. | |
Explanation | Use of the information, documents and data from the ECHA website is subject to the terms and conditions of this Legal Notice, and subject to other binding limitations provided for under applicable law, the information, documents and data made available on the ECHA website may be reproduced, distributed and/or used, totally or in part, for non-commercial purposes provided that ECHA is acknowledged as the source: "Source: European Chemicals Agency, http://echa.europa.eu/". Such acknowledgement must be included in each copy of the material. ECHA permits and encourages organisations and individuals to create links to the ECHA website under the following cumulative conditions: Links can only be made to webpages that provide a link to the Legal Notice page. | |
Synthesis routes and methods
Procedure details
Retrosynthesis Analysis
AI-Powered Synthesis Planning: Our tool employs the Template_relevance Pistachio, Template_relevance Bkms_metabolic, Template_relevance Pistachio_ringbreaker, Template_relevance Reaxys, Template_relevance Reaxys_biocatalysis model, leveraging a vast database of chemical reactions to predict feasible synthetic routes.
One-Step Synthesis Focus: Specifically designed for one-step synthesis, it provides concise and direct routes for your target compounds, streamlining the synthesis process.
Accurate Predictions: Utilizing the extensive PISTACHIO, BKMS_METABOLIC, PISTACHIO_RINGBREAKER, REAXYS, REAXYS_BIOCATALYSIS database, our tool offers high-accuracy predictions, reflecting the latest in chemical research and data.
Strategy Settings
Precursor scoring | Relevance Heuristic |
---|---|
Min. plausibility | 0.01 |
Model | Template_relevance |
Template Set | Pistachio/Bkms_metabolic/Pistachio_ringbreaker/Reaxys/Reaxys_biocatalysis |
Top-N result to add to graph | 6 |
Feasible Synthetic Routes
Disclaimer and Information on In-Vitro Research Products
Please be aware that all articles and product information presented on BenchChem are intended solely for informational purposes. The products available for purchase on BenchChem are specifically designed for in-vitro studies, which are conducted outside of living organisms. In-vitro studies, derived from the Latin term "in glass," involve experiments performed in controlled laboratory settings using cells or tissues. It is important to note that these products are not categorized as medicines or drugs, and they have not received approval from the FDA for the prevention, treatment, or cure of any medical condition, ailment, or disease. We must emphasize that any form of bodily introduction of these products into humans or animals is strictly prohibited by law. It is essential to adhere to these guidelines to ensure compliance with legal and ethical standards in research and experimentation.