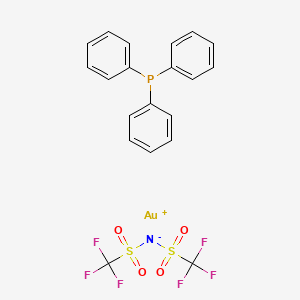
Bis(trifluoromethylsulfonyl)azanide;gold(1+);triphenylphosphane
- Click on QUICK INQUIRY to receive a quote from our team of experts.
- With the quality product at a COMPETITIVE price, you can focus more on your research.
Overview
Description
“Bis(trifluoromethylsulfonyl)azanide;gold(1+);triphenylphosphane” is a complex compound. The “Bis(trifluoromethylsulfonyl)azanide” part, also known as bistriflimide, is a non-coordinating anion with the chemical formula [(CF3SO2)2N]- . It is widely used in ionic liquids and is of importance in lithium-ion and lithium metal batteries . The compound also includes gold (1+) and triphenylphosphane.
Molecular Structure Analysis
The molecular structure of “this compound” is complex due to the presence of multiple components. The bis(trifluoromethylsulfonyl)azanide anion has a molecular formula of C2F6NO4S2 . The gold (1+) and triphenylphosphane components add further complexity to the structure.Physical And Chemical Properties Analysis
Bis(trifluoromethylsulfonyl)azanide has a boiling point of 190.5±50.0 °C at 760 mmHg and a vapor pressure of 0.5±0.4 mmHg at 25°C . It has 5 H bond acceptors and 1 H bond donor .Scientific Research Applications
Bis(trifluoromethylsulfonyl)azanide;gold(1+);triphenylphosphane has been used in a number of scientific research applications, including organic synthesis, catalysis, and biochemical and physiological studies. In organic synthesis, this compound has been demonstrated to be a highly effective catalyst for a number of organic reactions, including the synthesis of a variety of organic compounds. In catalysis, this compound has been used to catalyze the formation of carbon-carbon bonds and the hydrolysis of esters and amides. In biochemical and physiological studies, this compound has been used to investigate the mechanism of action and biochemical and physiological effects of various compounds.
Mechanism of Action
The mechanism of action of Bis(trifluoromethylsulfonyl)azanide;gold(1+);triphenylphosphane is not fully understood. However, it is believed that the gold(1+) center of the compound acts as a Lewis acid, which facilitates the formation of carbon-carbon bonds and the hydrolysis of esters and amides. Additionally, the trifluoromethylsulfonylazanide ligand is believed to act as a Lewis base, which stabilizes the gold(1+) center and facilitates the formation of carbon-carbon bonds.
Biochemical and Physiological Effects
The biochemical and physiological effects of this compound are not fully understood. However, it has been demonstrated to be a highly effective catalyst for a number of organic reactions, including the synthesis of a variety of organic compounds. Additionally, this compound has been used in a number of biochemical and physiological studies to investigate the mechanism of action and biochemical and physiological effects of various compounds.
Advantages and Limitations for Lab Experiments
The use of Bis(trifluoromethylsulfonyl)azanide;gold(1+);triphenylphosphane in laboratory experiments has a number of advantages. It is a highly efficient and selective catalyst for a variety of organic reactions, including the synthesis of a variety of organic compounds. Additionally, it is a relatively inexpensive and readily available compound. However, there are also some limitations to its use in laboratory experiments. This compound is sensitive to moisture and light, so it must be stored and handled carefully. Additionally, the reaction conditions must be carefully controlled to ensure the desired product is obtained.
Future Directions
The use of Bis(trifluoromethylsulfonyl)azanide;gold(1+);triphenylphosphane in scientific research is still in its early stages and there are a number of potential future directions for the compound. These include further investigations into its mechanism of action, the development of new synthetic methods using the compound, and the exploration of its potential applications in the pharmaceutical and biotechnological industries. Additionally, further research could be conducted into the biochemical and physiological effects of this compound, as well as its potential toxicity and environmental impact.
Synthesis Methods
The synthesis of Bis(trifluoromethylsulfonyl)azanide;gold(1+);triphenylphosphane involves the reaction of trifluoromethylsulfonylazanide (TFMSA) with gold(1+) and triphenylphosphane (TPP). This reaction is typically carried out at room temperature in an inert atmosphere such as nitrogen or argon. The reaction is usually carried out in the presence of a solvent such as acetonitrile or dimethylformamide. The reaction is typically complete within 24 hours. The product is then isolated by precipitation with aqueous methanol or other suitable solvent.
properties
{ "Design of the Synthesis Pathway": "The synthesis pathway for Bis(trifluoromethylsulfonyl)azanide;gold(1+);triphenylphosphane involves the reaction of gold(1+) with bis(trifluoromethylsulfonyl)azanide in the presence of triphenylphosphane.", "Starting Materials": [ "Gold(1+)", "Bis(trifluoromethylsulfonyl)azanide", "Triphenylphosphane" ], "Reaction": [ "Step 1: Dissolve gold(1+) in a suitable solvent such as dichloromethane.", "Step 2: Add bis(trifluoromethylsulfonyl)azanide to the gold(1+) solution and stir for several hours.", "Step 3: Add triphenylphosphane to the reaction mixture and stir for several more hours.", "Step 4: Isolate the product by filtration or other suitable means." ] } | |
CAS RN |
866395-16-6 |
Molecular Formula |
C20H15AuF6NO4PS2 |
Molecular Weight |
739.4 g/mol |
IUPAC Name |
bis(trifluoromethylsulfonyl)azanide;gold(1+);triphenylphosphane |
InChI |
InChI=1S/C18H15P.C2F6NO4S2.Au/c1-4-10-16(11-5-1)19(17-12-6-2-7-13-17)18-14-8-3-9-15-18;3-1(4,5)14(10,11)9-15(12,13)2(6,7)8;/h1-15H;;/q;-1;+1 |
InChI Key |
GJWZOHAPYGHXHP-UHFFFAOYSA-N |
SMILES |
C1=CC=C(C=C1)P(C2=CC=CC=C2)C3=CC=CC=C3.C(F)(F)(F)S(=O)(=O)[N-]S(=O)(=O)C(F)(F)F.[Au+] |
Canonical SMILES |
C1=CC=C(C=C1)P(C2=CC=CC=C2)C3=CC=CC=C3.C(F)(F)(F)S(=O)(=O)[N-]S(=O)(=O)C(F)(F)F.[Au+] |
Origin of Product |
United States |
Disclaimer and Information on In-Vitro Research Products
Please be aware that all articles and product information presented on BenchChem are intended solely for informational purposes. The products available for purchase on BenchChem are specifically designed for in-vitro studies, which are conducted outside of living organisms. In-vitro studies, derived from the Latin term "in glass," involve experiments performed in controlled laboratory settings using cells or tissues. It is important to note that these products are not categorized as medicines or drugs, and they have not received approval from the FDA for the prevention, treatment, or cure of any medical condition, ailment, or disease. We must emphasize that any form of bodily introduction of these products into humans or animals is strictly prohibited by law. It is essential to adhere to these guidelines to ensure compliance with legal and ethical standards in research and experimentation.