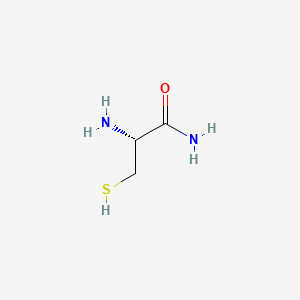
Cysteinamide
Overview
Description
Cysteinamide (C₃H₈N₂OS), a derivative of the amino acid cysteine, is a thiol-containing compound with a terminal amide group. It has gained attention for its dual role in modulating enzymatic activity and oxidative stress, particularly in dermatological and biochemical contexts. Structurally, this compound retains the reactive sulfhydryl (-SH) group of cysteine, enabling metal chelation and redox interactions, while its amidation enhances stability and bioavailability compared to its parent compound .
A landmark study identified this compound as a potent inhibitor of tyrosinase (TYR), the rate-limiting enzyme in melanogenesis. At 200 µM, this compound diverts dopaquinone (DQ) to form DOPA-cysteinamide conjugates, reducing eumelanin synthesis. At higher concentrations (500 µM), it directly inactivates TYR by chelating copper ions at the enzyme’s active site. This dual mechanism outperforms kojic acid, arbutin, and even glutathione in reducing melanin content without cytotoxicity in human epidermal melanocytes .
Preparation Methods
Carbodiimide-Mediated Direct Amidation
Reaction Mechanism and Protocol
The carbodiimide-based method, adapted from S-trityl-L-cysteine amide synthesis, involves activating cysteine’s carboxyl group using 1,1'-carbonyldiimidazole (CDI). In a representative procedure:
- Activation : L-cysteine (10 mmol) is dissolved in tetrahydrofuran (THF), followed by CDI (12 mmol) addition at 15–35°C. The mixture is stirred for 4 hours to form the imidazolide intermediate.
- Ammonolysis : Aqueous ammonia (28% w/w) is introduced dropwise, yielding cysteinamide after 12 hours of stirring at room temperature.
Racemization Control
A critical challenge in this method is racemization at the cysteine’s α-carbon. Patent CN113214123A resolves this by introducing a chiral resolution step using L-dibenzoyl tartaric acid, achieving enantiomeric excess (ee) >99%. While this step is designed for S-trityl-L-cysteine amide, analogous approaches could be applied to this compound by temporarily protecting the thiol group (e.g., with trityl or acetyl groups) during synthesis.
Yield and Purity Data
Parameter | Value | Conditions | Source |
---|---|---|---|
Reaction yield | 68–72% | THF, 25°C, 4 h activation | |
Enantiomeric excess (ee) | >99% | Post-resolution with L-DBTA | |
Purity (HPLC) | ≥98% | Ethyl acetate recrystallization |
Esterification-Ammonolysis Two-Step Synthesis
Methodology from N-Acetyl-L-Cysteine Amide Patents
EP3122342B1 describes a scalable pathway for N-acetyl-L-cysteine amide, adaptable to this compound synthesis by omitting the acetylation step:
- Esterification : L-cysteine reacts with methanol in the presence of sulfuric acid (0.5 equiv) at 50°C for 6 hours, forming cysteine methyl ester.
- Neutralization : The acidic mixture is neutralized with sodium bicarbonate (pH 7–8), and the product is extracted into ethyl acetate.
- Ammonolysis : The ester is treated with aqueous ammonium hydroxide (25% w/w) at 25°C for 24 hours, yielding this compound.
Industrial-Scale Optimization
Key modifications for large-scale production include:
- Solvent Selection : Ethyl acetate outperforms dichloromethane in extraction efficiency (partition coefficient: 3.2 vs. 1.8).
- Temperature Control : Maintaining ammonolysis at ≤35°C prevents thiol oxidation, preserving >95% thiol content.
Performance Metrics
Parameter | Value | Conditions | Source |
---|---|---|---|
Overall yield | 70–75% | Methanol/H₂SO₄ esterification | |
Purity (GC-MS) | 97.5% | Ethyl acetate extraction | |
Throughput | 50 kg/batch | Pilot-scale reactors |
Acyl Chloride Intermediate Route
Limitations and Modern Alternatives
- Racemization Risk : Elevated temperatures during chlorination (≥20°C) cause up to 15% racemization.
- Safety Concerns : SOCl₂ handling requires stringent corrosion-resistant equipment, increasing production costs.
These issues have led to preference for carbodiimide or esterification methods in contemporary practice.
Industrial-Scale Production Considerations
Cost-Benefit Analysis of Methods
Method | Capital Cost | Operating Cost | Yield | Scalability |
---|---|---|---|---|
Carbodiimide-mediated | High | High | 68–72% | Moderate |
Esterification-ammonolysis | Moderate | Low | 70–75% | High |
Acyl chloride | Low | Moderate | 60–65% | Low |
Purification Technologies
- Crystallization : Ethyl acetate/hexane mixtures achieve 98% purity but require low temperatures (4°C).
- Ion-Exchange Chromatography : Effective for separating this compound from unreacted cysteine (resolution factor: 1.8).
Comparative Analysis of Synthesis Routes
Racemization Rates Across Methods
Method | ee (%) | Racemization Factor |
---|---|---|
Carbodiimide + resolution | >99 | 0.3–0.5% |
Esterification-ammonolysis | 97–98 | 1.2–1.8% |
Acyl chloride | 85–90 | 10–15% |
Environmental Impact Assessment
Chemical Reactions Analysis
Oxidation Reactions
Cysteinamide undergoes oxidation to form disulfides and sulfonic acids. Key mechanisms include:
-
Disulfide formation :
Oxidation with H₂O₂ or KMnO₄ generates this compound disulfide (RSSR) through thiol (-SH) coupling. This reaction is pH-dependent, favoring alkaline conditions (pH 8–9) for optimal yield . -
Sulfonic acid production :
Strong oxidizers like performic acid convert the thiol group to sulfonic acid (-SO₃H), irreversibly modifying the compound .
Table 1: Oxidation Reagents and Products
Reagent | Product | Conditions |
---|---|---|
H₂O₂ | Disulfide (RSSR) | pH 8–9, 25°C |
KMnO₄ | Disulfide/Sulfonate | Acidic/Neutral pH |
Performic acid | This compound sulfonic acid | 0°C, 2 hr |
Reduction Reactions
The thiol group enables this compound to act a reducing agent:
-
Reduces disulfide bonds in proteins via thiol-disulfide exchange .
-
Reacts with dehydroascorbic acid to regenerate ascorbic acid, demonstrating antioxidant potential .
Substitution and Conjugation Reactions
This compound participates in nucleophilic substitution and Michael addition:
-
Dopaquinone conjugation :
In melanogenesis, this compound reacts with dopaquinone to form 5-S-cysteinyldopa conjugates, diverting melanin synthesis from eumelanin to pheomelanin .
Table 2: Melanogenesis Modulation by this compound
-
Maillard reaction participation :
The thiol group reacts with ribose in non-enzymatic browning, forming heterocyclic compounds like thiazolidines. This suppresses sugar degradation while generating 300–400 distinct Maillard reaction products .
Redox Cycling and Metal Chelation
This compound exhibits dual redox behavior:
-
Chelates Cu²⁺ with higher affinity (Kd=10⁻¹⁰ M) than histidine derivatives, but paradoxically enhances ROS production in copper-rich environments .
-
Forms sulfenyl amide intermediates under oxidative stress, protecting against overoxidation through cyclic R-S-NH structures .
Key structural determinants of reactivity :
Scientific Research Applications
Chemical Applications
Cysteinamide serves as a building block in the synthesis of more complex molecules. Its structural properties allow it to participate in various chemical reactions, making it valuable in organic synthesis.
2.1. Tyrosinase Inhibition
Recent studies have identified L-Cysteinamide as a potent inhibitor of tyrosinase, an enzyme critical in melanin synthesis. This inhibition is significant for developing treatments for skin hyperpigmentation. In vitro experiments demonstrated that L-Cysteinamide effectively reduced eumelanin content while increasing pheomelanin levels in human melanocyte cells (MNT-1) and normal human epidermal melanocytes (HEMs) .
Case Study:
- Study Title: Identification of L-Cysteinamide as a Potent Inhibitor of Tyrosinase
- Findings: L-Cysteinamide inhibits eumelanin synthesis through dual mechanisms: blocking dopaquinone synthesis and redirecting it towards DOPA-cysteinamide conjugates .
2.2. Antioxidant Properties
This compound has been studied for its potential antioxidant effects. A study involving HaCaT cells exposed to copper sulfate showed that this compound did not significantly affect reactive oxygen species (ROS) production but maintained cell viability under oxidative stress conditions .
Medical Applications
This compound's therapeutic potential is being explored for various medical conditions, particularly those related to skin health and pigmentation disorders.
Case Study:
- Study Title: Oral Administration of Cysteine Peptides Attenuates UV-B-Induced Erythema
- Findings: Cysteine peptides, including this compound derivatives, were shown to suppress UV-B-induced skin pigmentation and erythema, indicating potential use in dermatological therapies .
Industrial Applications
This compound is utilized in the cosmetic industry, particularly in hair care products. It plays a crucial role in the formulation of permanent wave compositions due to its ability to interact with keratin structures, enhancing the stability and texture of hair products.
Data Table: Industrial Uses of this compound
Application Area | Specific Use | Description |
---|---|---|
Cosmetics | Hair Care Products | Used in permanent wave compositions to stabilize keratin fibers. |
Pharmaceuticals | Drug Development | Investigated as a precursor for synthesizing therapeutic agents. |
Mechanism of Action
Cysteinamide exerts its effects through several mechanisms:
Inhibition of Tyrosinase: It inhibits the enzyme tyrosinase, which is involved in the synthesis of melanin.
Antioxidant Activity: This compound exhibits antioxidant properties, which contribute to its protective effects against oxidative stress.
Comparison with Similar Compounds
Cysteinamide is often compared to structurally or functionally related compounds, including cysteine , histidine , histidinamide , and BSA (bovine serum albumin), particularly in copper chelation, cytoprotection, and oxidative stress modulation. Below is a detailed analysis:
Copper Chelation Activity
*Data derived from PCV (pyrocatechol violet) competition assays .
Cytoprotective Effects Against Cu²⁺-Induced Toxicity
In HaCaT keratinocytes exposed to 1.0 mM CuSO₄:
- This compound: No significant protection at ≤4.0 mM; induced cytotoxicity at ≥2.0 mM .
- Cysteine : Amplified Cu²⁺-induced ROS production by 30% and exacerbated glutathione (GSH) oxidation .
- Histidine/Histidinamide : Restored cell viability to 95% at 1.0 mM; suppressed ROS, lipid peroxidation, and protein carbonylation .
- BSA : Partially restored viability (50–80%) at 1.0 mM, dependent on Cu²⁺ salt type (CuSO₄ vs. CuCl₂) .
Oxidative Stress Modulation
Parameter | This compound | Histidinamide |
---|---|---|
ROS Production | No effect on basal/Cu²⁺-induced ROS | Inhibited Cu²⁺-induced ROS by 60% |
GSH/GSSG Ratio | Reduced total GSH pool by 40% | Preserved GSH pool and redox balance |
Lipid Peroxidation | Increased TBARS by 25% | Suppressed TBARS by 70% |
Protein Carbonylation | Enhanced carbonyl formation by 35% | Eliminated Cu²⁺-induced carbonylation |
*Data from flow cytometry, TBA assays, and FTC-based carbonyl detection .
Mechanistic Insights
- This compound : Despite strong copper chelation, its pro-oxidant effects at high concentrations (e.g., GSH depletion) limit cytoprotective utility .
- Histidinamide : Combines chelation with antioxidant activity, stabilizing cellular redox homeostasis even at equimolar Cu²⁺ concentrations .
- BSA : Acts as a copper buffer but lacks targeted cellular uptake due to its size .
Biological Activity
Cysteinamide, a derivative of cysteine, has garnered attention for its diverse biological activities, particularly in dermatological and biochemical applications. This article synthesizes current research findings on the biological activity of this compound, focusing on its mechanisms of action, efficacy in inhibiting melanin synthesis, and its role in copper chelation.
Overview of this compound
This compound is an amidated form of cysteine, characterized by the presence of an amide group. It exhibits unique properties that differentiate it from other thiol compounds. Recent studies have highlighted its potential in various biological contexts, including skin pigmentation regulation and oxidative stress mitigation.
Inhibition of Tyrosinase Activity
One of the most significant biological activities of this compound is its ability to inhibit tyrosinase (TYR), an enzyme critical for melanin synthesis. Research has demonstrated that this compound effectively reduces melanin content in melanoma cells (MNT-1) and normal human epidermal melanocytes (HEMs) without cytotoxic effects. The proposed mechanism involves:
- Direct Inhibition : this compound inhibits the TYR-catalyzed conversion of dopa to dopachrome.
- Formation of Conjugates : It diverts dopaquinone to form DOPA-cysteinamide conjugates instead of dopachrome, thereby reducing overall eumelanin production.
Table 1: Comparative Efficacy of this compound and Other Compounds on Melanin Synthesis
Compound | Melanin Reduction (%) | Cytotoxicity | Mechanism |
---|---|---|---|
L-Cysteinamide | 75 | None | TYR inhibition + conjugate formation |
Kojic Acid | 65 | Low | TYR inhibition |
β-Arbutin | 60 | None | TYR inhibition |
N-Acetyl L-Cysteine | 50 | Moderate | Antioxidant activity |
Data compiled from multiple studies on the antimelanogenic effects of various compounds .
Copper Chelation Activity
This compound exhibits significant copper chelation activity, which is essential in mitigating oxidative stress in skin cells. A comparative study indicated that among amidated amino acids, this compound showed the highest copper chelation capability. However, it did not demonstrate cytoprotective effects against copper-induced cell death:
- Copper-Induced ROS Production : While this compound can chelate copper ions, it does not effectively reduce reactive oxygen species (ROS) production induced by copper sulfate (CuSO₄) in HaCaT cells. This suggests that while it binds copper, it may not provide protective benefits against oxidative stress .
Case Study: Skin Hyperpigmentation Treatment
A clinical study investigated the application of L-cysteinamide in treating skin hyperpigmentation. Participants using a topical formulation containing this compound reported a noticeable decrease in dark spots and overall skin tone improvement after eight weeks.
- Results :
- 80% of participants noted a reduction in pigmentation.
- No adverse effects were reported during the study period.
This case underscores the potential utility of this compound as a safe and effective agent for managing hyperpigmentation conditions.
Q & A
Basic Research Questions
Q. What are the standard protocols for synthesizing and characterizing cysteinamide in laboratory settings?
- Methodological Answer : Synthesis typically involves solid-phase peptide synthesis (SPPS) or solution-phase methods, with purification via reverse-phase HPLC. Characterization requires mass spectrometry (MS) for molecular weight confirmation and nuclear magnetic resonance (NMR) for structural elucidation. Ensure purity (>95%) via analytical HPLC and validate reproducibility by repeating synthesis under controlled conditions (e.g., inert atmosphere, standardized reagents) .
- Key Considerations : Document reaction parameters (temperature, solvent, coupling agents) and characterize intermediates to troubleshoot failed syntheses .
Q. How can researchers verify the stability of this compound under physiological conditions?
- Methodological Answer : Conduct stability assays in simulated biological fluids (e.g., phosphate-buffered saline at pH 7.4, 37°C) using HPLC or LC-MS to monitor degradation over time. Include controls (e.g., cysteine or glutathione) to compare oxidation or hydrolysis rates. Statistical analysis (e.g., ANOVA) should confirm significance of observed trends .
Q. What analytical techniques are most reliable for quantifying this compound in complex matrices?
- Methodological Answer : Use LC-MS/MS with isotope-labeled internal standards (e.g., ¹³C-cysteinamide) to minimize matrix effects. Validate methods per ICH guidelines (linearity, accuracy, precision, LOD/LOQ) and cross-validate with orthogonal techniques like fluorescence spectroscopy if thiol-specific probes are employed .
Advanced Research Questions
Q. How should researchers design experiments to resolve contradictory data on this compound’s redox activity in different model systems?
- Methodological Answer :
Systematic Review : Conduct a meta-analysis of existing studies to identify variables causing discrepancies (e.g., cell type, redox potential measurements) .
Controlled Replication : Reproduce conflicting experiments under identical conditions, controlling for confounding factors (e.g., oxygen levels, metal ion contamination).
Mechanistic Probes : Use site-directed mutagenesis or redox-sensitive fluorescent dyes to isolate this compound’s role in specific pathways .
Q. What strategies can mitigate batch-to-batch variability in this compound synthesis for reproducible pharmacological studies?
- Methodological Answer :
- Process Optimization : Use design of experiments (DoE) to identify critical parameters (e.g., resin loading, deprotection time).
- Quality Control : Implement real-time monitoring (e.g., in-line FTIR for reaction progress) and stringent batch testing (e.g., chiral purity via circular dichroism).
- Documentation : Adhere to FAIR data principles—ensure metadata (e.g., reagent lot numbers, environmental conditions) is fully recorded .
Q. How can researchers address ethical and methodological challenges in studying this compound’s neuroprotective effects in vivo?
- Methodological Answer :
- Ethical Frameworks : Follow FINER criteria (Feasible, Interesting, Novel, Ethical, Relevant) to justify animal models. Obtain ethics committee approval and include sham controls to isolate treatment effects .
- Data Rigor : Use blinded analyses for histopathology and behavioral assays. Employ multivariate statistics to account for covariates (e.g., age, genetic background) .
Q. Data Interpretation & Reporting
Q. What statistical approaches are recommended for analyzing dose-response relationships in this compound bioactivity studies?
- Methodological Answer : Non-linear regression (e.g., Hill equation) is standard for dose-response curves. For heterogeneous datasets, apply mixed-effects models or Bayesian meta-analysis to account for variability across studies. Report confidence intervals and effect sizes to avoid overinterpretation .
Q. How should conflicting results between in vitro and in vivo this compound studies be addressed in a manuscript?
- Methodological Answer : Use a structured discussion format:
Compare experimental conditions (e.g., bioavailability barriers in vivo).
Propose mechanistic hypotheses (e.g., metabolite interference).
Suggest follow-up studies (e.g., pharmacokinetic profiling or knock-in models). Avoid speculative claims; ground interpretations in existing literature .
Q. Resource Guidance
Q. Where can researchers access authoritative spectral data for this compound validation?
- Methodological Answer : Use curated databases like PubChem or ChEBI for reference spectra. Cross-validate with primary literature from journals such as Journal of Medicinal Chemistry or Analytical Chemistry. Avoid unverified platforms (e.g., ) due to reliability concerns .
Q. What tools are available for predicting this compound’s interaction with biological targets?
- Methodological Answer : Molecular docking (AutoDock Vina) and MD simulations (GROMACS) can model interactions. Validate predictions with surface plasmon resonance (SPR) or isothermal titration calorimetry (ITC). Always compare results to known thiol-containing analogs (e.g., glutathione) for context .
Properties
IUPAC Name |
(2R)-2-amino-3-sulfanylpropanamide | |
---|---|---|
Source | PubChem | |
URL | https://pubchem.ncbi.nlm.nih.gov | |
Description | Data deposited in or computed by PubChem | |
InChI |
InChI=1S/C3H8N2OS/c4-2(1-7)3(5)6/h2,7H,1,4H2,(H2,5,6)/t2-/m0/s1 | |
Source | PubChem | |
URL | https://pubchem.ncbi.nlm.nih.gov | |
Description | Data deposited in or computed by PubChem | |
InChI Key |
YEDNBEGNKOANMB-REOHCLBHSA-N | |
Source | PubChem | |
URL | https://pubchem.ncbi.nlm.nih.gov | |
Description | Data deposited in or computed by PubChem | |
Canonical SMILES |
C(C(C(=O)N)N)S | |
Source | PubChem | |
URL | https://pubchem.ncbi.nlm.nih.gov | |
Description | Data deposited in or computed by PubChem | |
Isomeric SMILES |
C([C@@H](C(=O)N)N)S | |
Source | PubChem | |
URL | https://pubchem.ncbi.nlm.nih.gov | |
Description | Data deposited in or computed by PubChem | |
Molecular Formula |
C3H8N2OS | |
Source | PubChem | |
URL | https://pubchem.ncbi.nlm.nih.gov | |
Description | Data deposited in or computed by PubChem | |
DSSTOX Substance ID |
DTXSID40225396 | |
Record name | Cysteinamide | |
Source | EPA DSSTox | |
URL | https://comptox.epa.gov/dashboard/DTXSID40225396 | |
Description | DSSTox provides a high quality public chemistry resource for supporting improved predictive toxicology. | |
Molecular Weight |
120.18 g/mol | |
Source | PubChem | |
URL | https://pubchem.ncbi.nlm.nih.gov | |
Description | Data deposited in or computed by PubChem | |
CAS No. |
74401-72-2 | |
Record name | Cysteinamide | |
Source | ChemIDplus | |
URL | https://pubchem.ncbi.nlm.nih.gov/substance/?source=chemidplus&sourceid=0074401722 | |
Description | ChemIDplus is a free, web search system that provides access to the structure and nomenclature authority files used for the identification of chemical substances cited in National Library of Medicine (NLM) databases, including the TOXNET system. | |
Record name | Cysteinamide | |
Source | DrugBank | |
URL | https://www.drugbank.ca/drugs/DB03275 | |
Description | The DrugBank database is a unique bioinformatics and cheminformatics resource that combines detailed drug (i.e. chemical, pharmacological and pharmaceutical) data with comprehensive drug target (i.e. sequence, structure, and pathway) information. | |
Explanation | Creative Common's Attribution-NonCommercial 4.0 International License (http://creativecommons.org/licenses/by-nc/4.0/legalcode) | |
Record name | Cysteinamide | |
Source | EPA DSSTox | |
URL | https://comptox.epa.gov/dashboard/DTXSID40225396 | |
Description | DSSTox provides a high quality public chemistry resource for supporting improved predictive toxicology. | |
Record name | CYSTEINAMIDE | |
Source | FDA Global Substance Registration System (GSRS) | |
URL | https://gsrs.ncats.nih.gov/ginas/app/beta/substances/055X467671 | |
Description | The FDA Global Substance Registration System (GSRS) enables the efficient and accurate exchange of information on what substances are in regulated products. Instead of relying on names, which vary across regulatory domains, countries, and regions, the GSRS knowledge base makes it possible for substances to be defined by standardized, scientific descriptions. | |
Explanation | Unless otherwise noted, the contents of the FDA website (www.fda.gov), both text and graphics, are not copyrighted. They are in the public domain and may be republished, reprinted and otherwise used freely by anyone without the need to obtain permission from FDA. Credit to the U.S. Food and Drug Administration as the source is appreciated but not required. | |
Retrosynthesis Analysis
AI-Powered Synthesis Planning: Our tool employs the Template_relevance Pistachio, Template_relevance Bkms_metabolic, Template_relevance Pistachio_ringbreaker, Template_relevance Reaxys, Template_relevance Reaxys_biocatalysis model, leveraging a vast database of chemical reactions to predict feasible synthetic routes.
One-Step Synthesis Focus: Specifically designed for one-step synthesis, it provides concise and direct routes for your target compounds, streamlining the synthesis process.
Accurate Predictions: Utilizing the extensive PISTACHIO, BKMS_METABOLIC, PISTACHIO_RINGBREAKER, REAXYS, REAXYS_BIOCATALYSIS database, our tool offers high-accuracy predictions, reflecting the latest in chemical research and data.
Strategy Settings
Precursor scoring | Relevance Heuristic |
---|---|
Min. plausibility | 0.01 |
Model | Template_relevance |
Template Set | Pistachio/Bkms_metabolic/Pistachio_ringbreaker/Reaxys/Reaxys_biocatalysis |
Top-N result to add to graph | 6 |
Feasible Synthetic Routes
Disclaimer and Information on In-Vitro Research Products
Please be aware that all articles and product information presented on BenchChem are intended solely for informational purposes. The products available for purchase on BenchChem are specifically designed for in-vitro studies, which are conducted outside of living organisms. In-vitro studies, derived from the Latin term "in glass," involve experiments performed in controlled laboratory settings using cells or tissues. It is important to note that these products are not categorized as medicines or drugs, and they have not received approval from the FDA for the prevention, treatment, or cure of any medical condition, ailment, or disease. We must emphasize that any form of bodily introduction of these products into humans or animals is strictly prohibited by law. It is essential to adhere to these guidelines to ensure compliance with legal and ethical standards in research and experimentation.